Research projects
Ongoing projects
Morpho- and habitat dynamics
- The Influence of Morphodynamic Processes on Fluvial Habitat Dynamics
- Modelling floating matter in rivers
- Stability of alpine rivers
- Sustainable storage hydropower for a resilient future energy system
- Composite Modelling of Dam Breaching due to Overtopping
- Morphodynamic 1-D modelling of the future development of the Alpine Rhine estuary
- Suspended sediment transport interaction with vegetation on floodplains
- Feasibility study for numerical modelling of large sediment tributaries
- Numerical Modelling of Mesohabitats
- Eco-morphodynamic modelling for gravel bed rivers (BASEveg)
- Sediment budget analysis and management in the Ötztal
- Two dimensional depth-averaged modelling of braided gravel-bed rivers
Hydraulic structures
- Safe design of hydraulic structures for high energy air-water flows (SAFAIR - part B)
- HydroLEAP – Downstream fish movement at Massongex-Bex-Rhone Demonstrator
- 3-D modeling of Aare run-of-river power plants for planning of fish migration measures
- Fish downstream migration at-run-off-river hydropower plant Wildegg-Brugg
- Curved-Bar Rack (CBR) at hydropower plant Herrentöbeli (Thur)
Risk assessment and flood protection
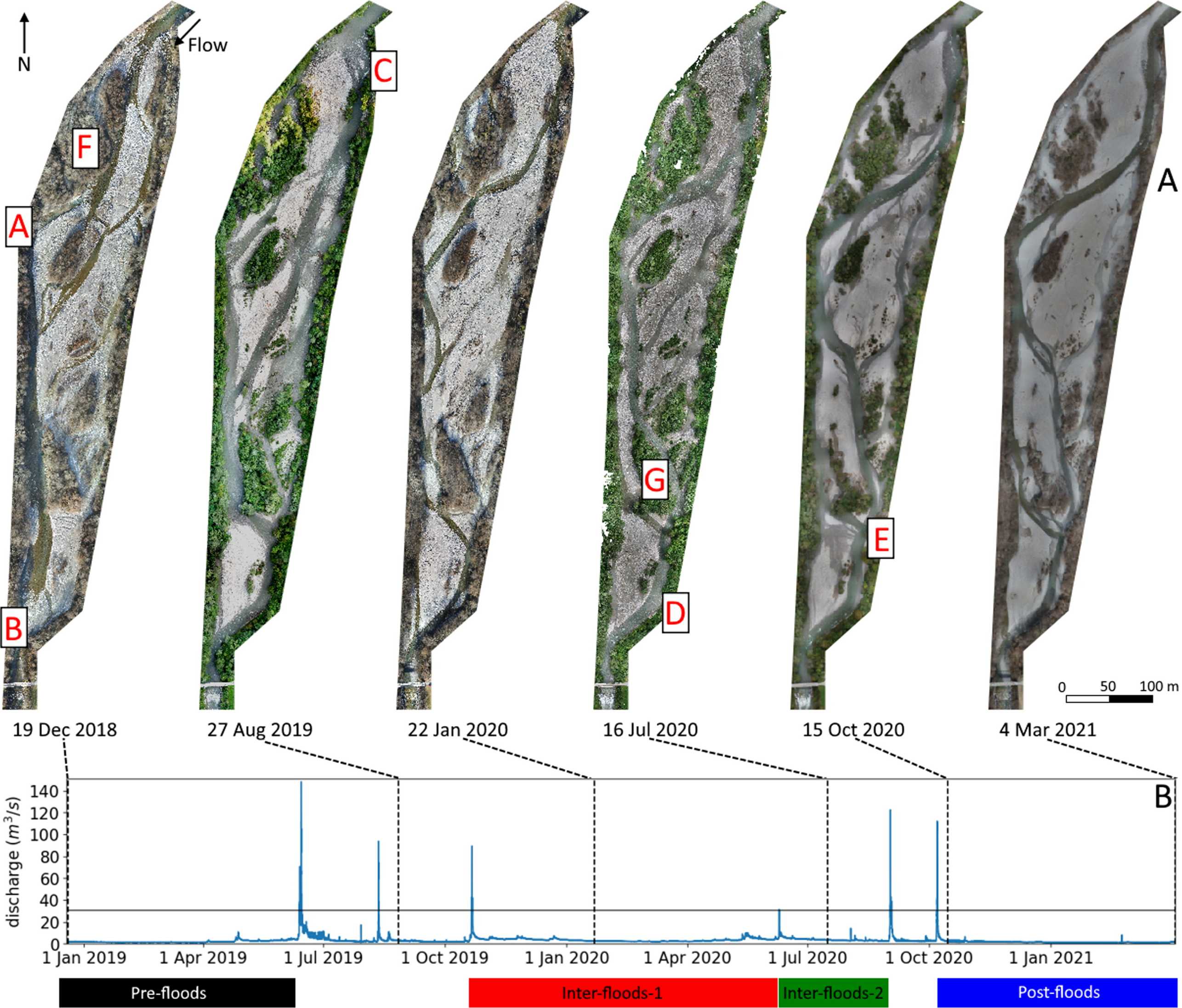
The need to quantify fluvial habitat has been increasingly recognized as essential for effective river management and conservation. However, the influence of morphodynamic processes on the quantity and quality of habitat have not yet been fully clarified. Sediment dynamics, i.e. sediment transport, erosion, and deposition, play a critical role in shaping the physical characteristics of river systems, which in turn can impact the distribution and quality of fluvial habitats.
The aim of this research project is to investigate the relationships between morphodynamic processes and fluvial habitat dynamics, specifically focusing on the effects of bedload supply and hydrological boundary conditions on habitat characteristics at the reach scale. The research will also explore the dynamics and persistence of habitat refugia during natural disturbances such as floods.
To achieve this aim, the research will tackle the following research questions:
• How habitat distribution changes with discharge? And how do habitats change due to variable river morphology?
• What is the relationship between river morphology and habitat persistence?
• What are the morphological requirements for habitats to be maintained or destroyed during a flood?
The study will utilize numerical models to simulate morphological changes and determine habitat characteristics in river sections with pronounced morphological changes. The research will investigate the relationship between habitat dynamics and morphological changes during different boundary conditions (discharge and bedload supply) and for different river morphologies (topography of the river bed). Overall, this research aims to provide a better understanding of the relationship between morphodynamic processes and fluvial habitat dynamics, which can help support effective river management and conservation efforts.
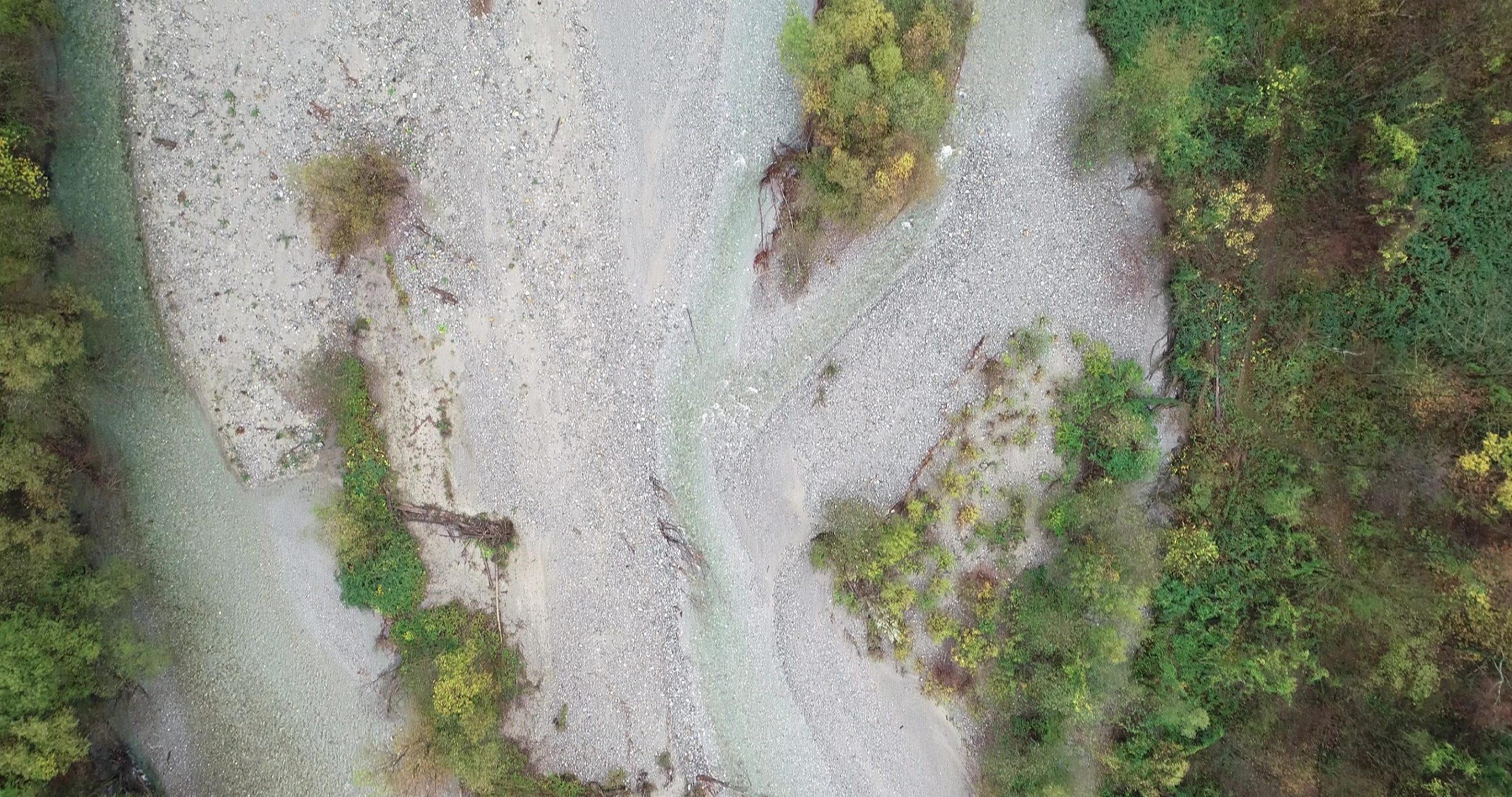
The transport and deposition of floating matter in rivers, such as plant seeds, wood, and organic debris, are crucial components of river ecosystems. In particular, plant seeds that are transported by water (i.e. hydrochory) allow plants colonizing new areas and maintaining biodiversity along river channels and floodplains. Seeds are often transported on the water surface, and they are deposited when the water level recedes, creating typical strip-like patterns along channels. It is well known that flow velocity has a great influence on deposition pattern and travel distances of seeds. However, a quantitative understanding of the specific role of river hydro-morphology on mediating hydrochory remains very limited.
The goal of this project is to investigate the relationship between river hydro-morphology and seed dispersal and deposition. In this scope, we plan to develop a new Lagrangian approach that allows us to track particle trajectories through a flow field. The model will be integrated within the BASEMENT software, which solves the two-dimensional equations for the water flow. The new approach will be designed in a way that can be used to simulate the transport of different kinds of floating objects from massless particles to larger debris. The model development will be complemented with laboratory and field experiments to produce a dataset for calibration and validation of the model. Laboratory experiments will be conducted in a hydraulic flume to test floating properties of different particle sizes and shapes, including seeds of different species and artificial mimics. The scope of field experiments will be then to investigate the deposition patterns of artificial mimics on a real river morphology under different discharges. This will be done on a braided reach of the Moesa river, Switzerland.
The expected output of the project will provide a quantitative estimate of the role of river hydro-morphology on particle transport and deposition. By focusing on plant seeds, we will be able to shed light on the role of hydrochory in riparian vegetation dynamics and support conservation measures of endangered riparian plant species in Switzerland.
This study is part of the research program “Hydraulic engineering and ecology” in the phase 2022-2026 and is co-financed by the Swiss Federal Office for the Environment (FOEN).
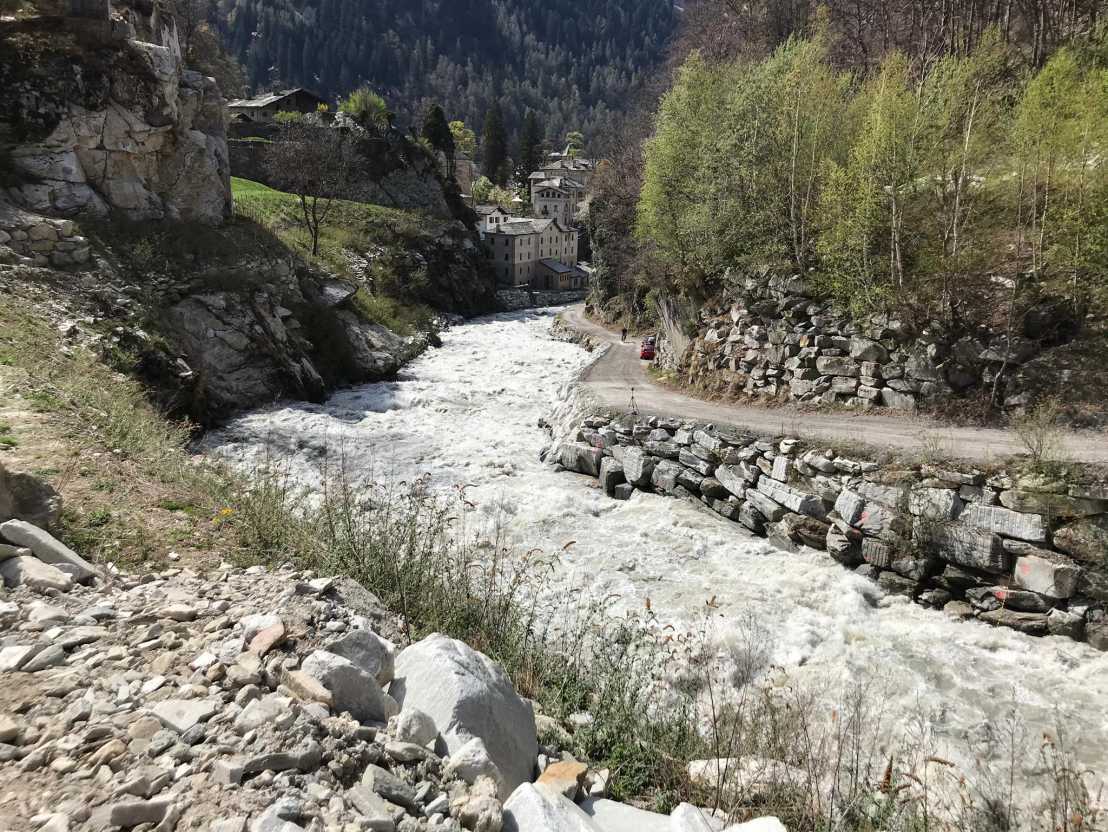
Alpine rivers are ecologically valuable and provide energy as well as recreational benefits to the public but also pose risks in case of floodings and bed failures. In the past, large artificial (concrete) structures were used to reduce the damage caused during such events. Nowadays such structures are not in agreement with the Swiss Waters Protection Act and more natural-like solutions have to be developed. One promising approach is the systematic placement of large roughness elements such as boulders in the rivers to strengthen the river bed and increase the flow resistance (e.g. unstructured block ramps). Although such structures already exist there is little knowledge about the exact flow field and forces acting around and on the roughness elements.
This project aims to give more insight into the hydromorphological processes in alpine rivers. Using highly resolved 3D-numerical simulations, the flow field can be investigated throughout the whole domain. This includes the estimation of the flow velocities in the water, the shear stresses on the river bed as well as instantaneous and averaged forces acting on the macro roughness elements. By altering parameters such as boulder density, slope or discharge, their influence on the stability of alpine rivers can be investigated.
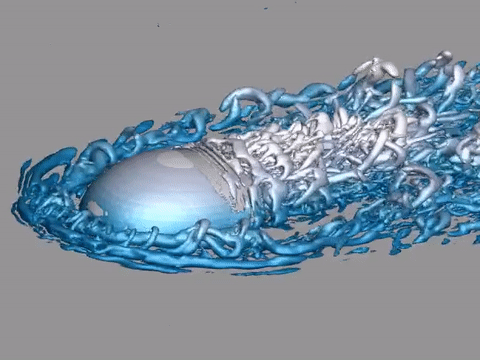
The workflow is separated into three main parts. In a first step, different numerical models will be evaluated using simple cases well-described in literature. In the second step, more complex scenarios will be investigated using multiple roughness elements and nature-like river topography. The validation data comes mainly from laboratory experiments. In the third step, the topography of Swiss rivers will be used to estimate the stability.
Reservoir sedimentation has been recognized to be one of the factors having negative impact on hydropower storage capacities in Switzerland. In 2019, the Swiss Federal Office of Energy has published an updated edition of its hydropower potential study, stating that considerable efforts are necessary to maintain and extend today’s hydropower capacities to meet the requirements set out in the Swiss Energy Strategy 2050. It has been anticipated that reservoir sedimentation will cause a loss of about 7% of seasonal storage capacity in Swiss reservoirs until 2050 (since 2019). However, this estimation is just an average value and is subject to considerable uncertainties. In many reservoirs, the sedimentation rate is much more acute, creating operational and dam safety problems.
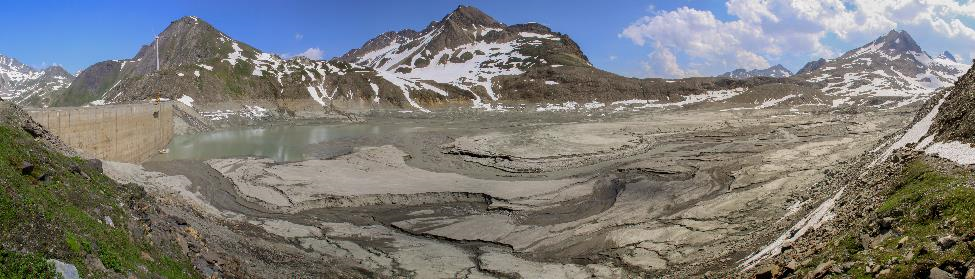
This research project aims at reducing the uncertainties related to the assessment of long-term reservoir sedimentation under the effect of climate change; and also aims to investigate different sediment management strategies as countermeasures. Two Swiss reservoirs will be selected as case study sites in this research. Numerical modelling will be applied using the BASEMENT software to simulate the reservoir sedimentation process and to assess the effectiveness of different management strategies. Field measurement campaigns will be conducted to acquire the data related to hydrology, topography and sediment transport related parameters that are essential for model development.
The outcome of this research is expected to provide better information of the future availability of reservoir storage capacities, and also the development of a conceptual framework for the application of different countermeasures against reservoir sedimentation.
This project is a part of the external page RESPONSE Doctoral Program. The hydropower section of the external page Swiss Federal Office of Energy is involved as partner organization and a secondment is planned during the second half of the project.

During a major riverine or coastal flood event, the water level at a dam or dike can exceed the top elevation of the structure. This causes “overtopping” flow, which sends water flowing along the downstream side of the structure. In the case of earthen embankments, this flow can cause erosion of the embankment material (often sand, gravel, or rock). Given enough time, the erosion can carry away a significant portion of the structure, thereby creating a “breach” that allows uncontrolled outflow through the opening.
Many earthen embankments consist of a single material type throughout the entire structure. These are known as “homogeneous” embankments. The overtopping breaching processes of homogeneous embankments have been well-studied, including multiple past projects at VAW. However, many embankments have multiple layers of material, each with a specific purpose: such as structural integrity, seepage control, and erosion control. These are known as “zoned” embankments, and there has been very limited study of the breaching of these types of structures. The goal of this project is to investigate the formation and development of breaches caused by the overtopping of zoned embankments.
First, physical experiments will be performed in the VAW laboratory. Scale-model embankments will be built in a hydraulic flume. The embankments will be overtopped, and the resulting breach development will be captured by a network of cameras and a photogrammetric data analysis software, making use of a structured grid that is projected on the embankment’s surface by a beamer.
Second, a numerical model of embankment breaching will be developed with VAW’s BASEMENT software. With these two modelling strategies (physical and numerical) and their systematic combination (composite modelling), we will model dozens of different dam designs, by varying both hydraulic and key design parameters. The latter parameters include embankment size, material type, number of layers, thickness of layers, and type of seepage control.
Third, a probabilistic framework will be used to quantify the impact of different parameters on breach formation. Based on this, we plan to develop simple equations and a parametric model that will allow for quick estimation of breach processes and results. These include the maximum discharge through the breach, the rate of breach growth, and failure modes. We will compare these results for the different types of embankments (homogeneous vs. zoned).
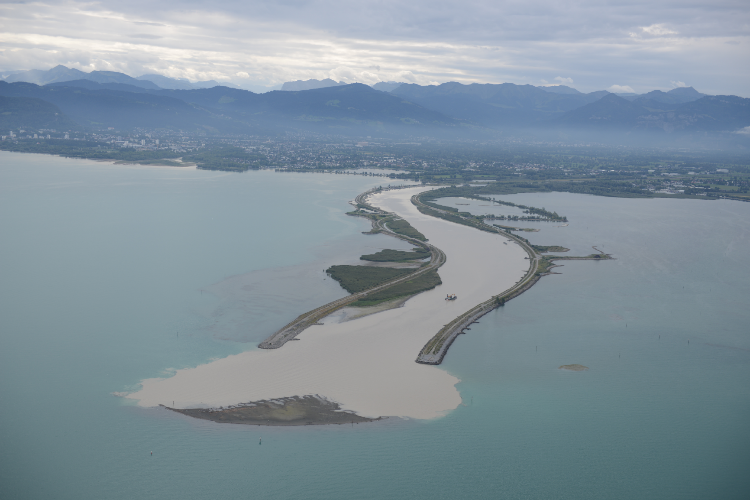
In order to prevent the aggradation of the shallower bays around the new Rhine delta in the east-ern part of Lake Constance, dams were built successively to prolong the estuary into the lake and thus convey the transported sediment to deeper parts of the lake to settle. The estuary dams have meanwhile reached their planned extent of about 4 km length.
In 2018, low water levels in the lake revealed sediment depositions at the end of the estuary (see Fig. 1). This demonstrated the necessity to be able to gauge the aggradation dynamics of the estuary and long-term developments.
In the scope of this project, a 1-D morphodynamic model is used to acquire an understanding of the underlying and long-term aggradation and erosion processes within the estuary. The objective is to establish the maximum length of the estuary without impairment of the upstream river regulation and whether this length has been reached yet. Furthermore, it is to be investigated, how gravel extraction is influencing the processes and developments in the estuary and vice versa.
For this analysis, 1-D morphodynamic simulations are being performed using the software BASEMENT.
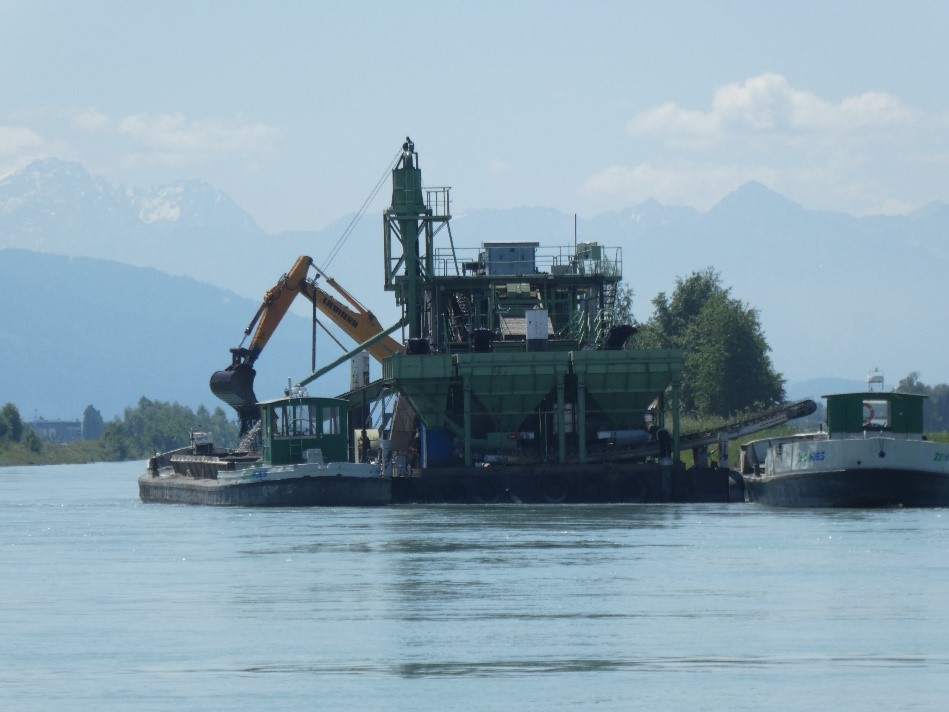
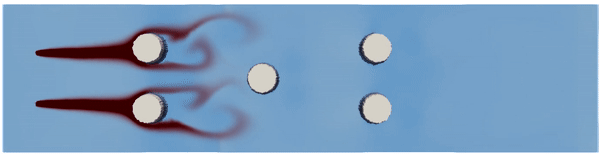
Riverine environments are amongst the most complex ecosystems on the planet. With several anthropogenic factors increasingly disrupting natural fluvial dynamics, particularly through regularization, the need for re-establishing the ecological properties of these systems has gained relevance in the last decade.
Of particular interest are the floodplains along the river network, primarily for safety against floods, which comprise an extensive realm for restoring ecological functions and establishing new habitats for various species.
However, floodplain vegetation may increase the deposition of suspended sediments and reduce flow conveyance during floods. Considering the central role of rivers in landlocked territories with high development, like Switzerland, the success of river restoration efforts depends greatly on state-of-the-art knowledge, stakeholder cooperation and reliable operational tools for river management and restoration.
The main goal of this study is to contribute to the understanding of the involved processes and answer the following questions:
- How much fine sediment is deposited during floods?
- Which vegetation properties have greater effects on suspended sediment transport and deposition?
- To which extent can 2D numerical models successfully simulate deposition-erosion patterns?
A numerical hydro-morphodynamic 2D model based at VAW (BASEMENT) is herein extended with turbulence and suspended sediment solving capabilities. The implementation follows a High-Performance Computing paradigm, to deliver on the workloads imposed by the spatial and temporal scales required in modelling sediment transport and deposition. Laboratory measurements will provide the support for physical validation while applications to Swiss rivers are planned as case-studies for showcasing the benefits of the developed features.
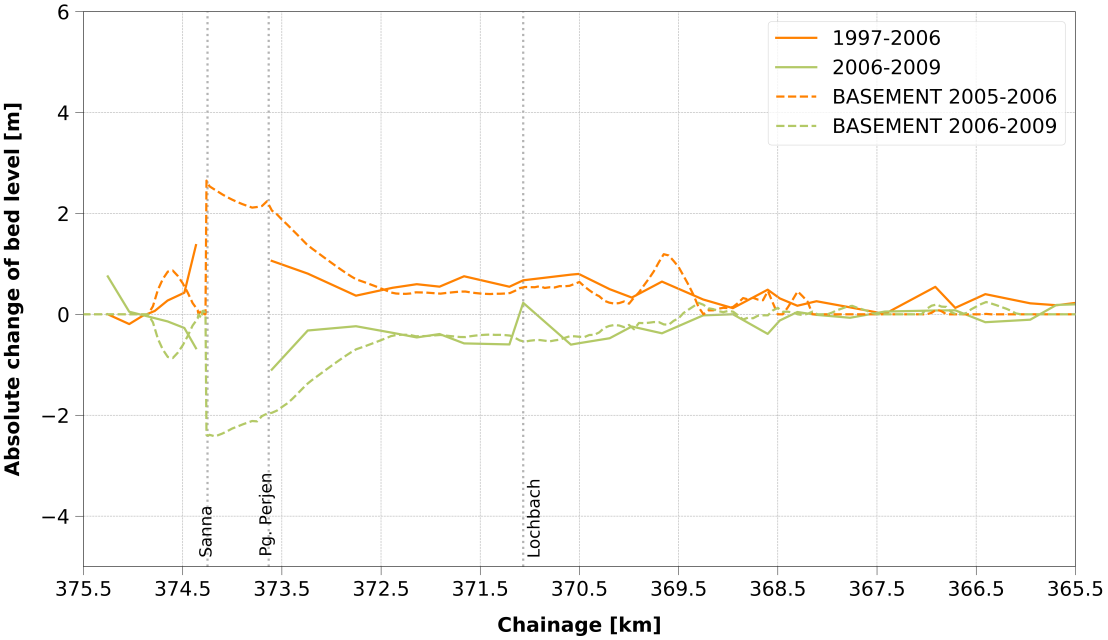
During the flood event of August 2005, the Inn river received an estimated sediment volume of 300’000 m3 from its tributary Sanna. A considerable part of the sediment was deposited in the vicinity of the confluence, resulting in significant aggradation of several meters and increased sediment transport further downstream.
The numerical modelling of lateral sediment input of this magnitude and the related aggradation and erosion processes is not trivial. By means of a feasibility study, it was investigated whether and how the morphological processes at the Inn, related to the sediment input from the Sanna, can be simulated. Therefore, one-dimensional hydro- and morphodynamic simulations were carried out with the software BASEMENT.
The investigations revealed that modelling such a large sediment input as a lateral source is feasible if the input is distributed over three or more cross sections. Cross section intervals of 10 m within 100 m upstream and downstream of the confluence and intervals of 50 m for the remaining model perimeter proved to be sufficient in the present case. Furthermore, a multigrain representation of the sediment with a mean diameter of 35 mm together with the sediment transport formula of Hunziker (1995) resulted in good agreement between simulated and measured bed level changes over time.
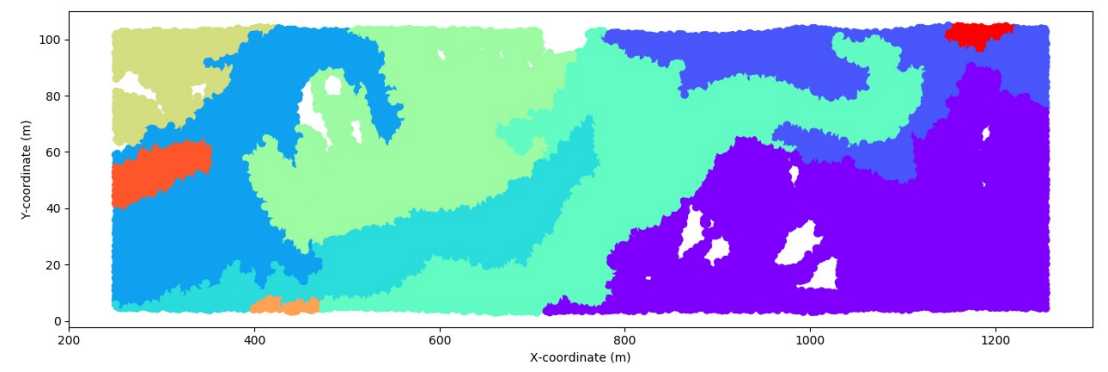
Many rivers have been adapted for flood protection and navigability in the past. In many cases these adaptations have had detrimental effects on the biota living in and around the river by destroying or damaging their habitat.
In recent years increasingly more attention is given to the harmful effects of climate change on freshwater habitat and the focus of river project design has shifted to also include ecological aspects. A fast and objective method to classify areas of ecological fitness on the right spatial scales could aid in these projects and evaluate the effects climate change or management decisions could have on lotic species.
This project aims to develop such a method. The spatial scale will be the mesoscale, which assumes the habitat use of individuals is not only dependent on local parameters but also those of its intermediate surroundings. This scale is easiest to interpret and use in river restoration projects. Several of these methods, like for instance external page http://www.mesohabsim.org already exist. We will aim to expand these methods.
In this project the use of a numerical hydromorphodynamic model (BASEMENT) will be used to obtain spatially and temporally varying data. Based on this data, an unsupervised algorithm will be used to identify mesohabitats (zones of equal habitat suitability) for various species. The primary focus will be on fish species, but invertebrates and other lotic species will also be considered. This unsupervised approach should ensure that the method will be fast and objective, an additional benefit will be that longer reaches can be analyzed.
Field measurements in Swiss rivers are planned. The results from these measurements will be compared to the model outcomes.
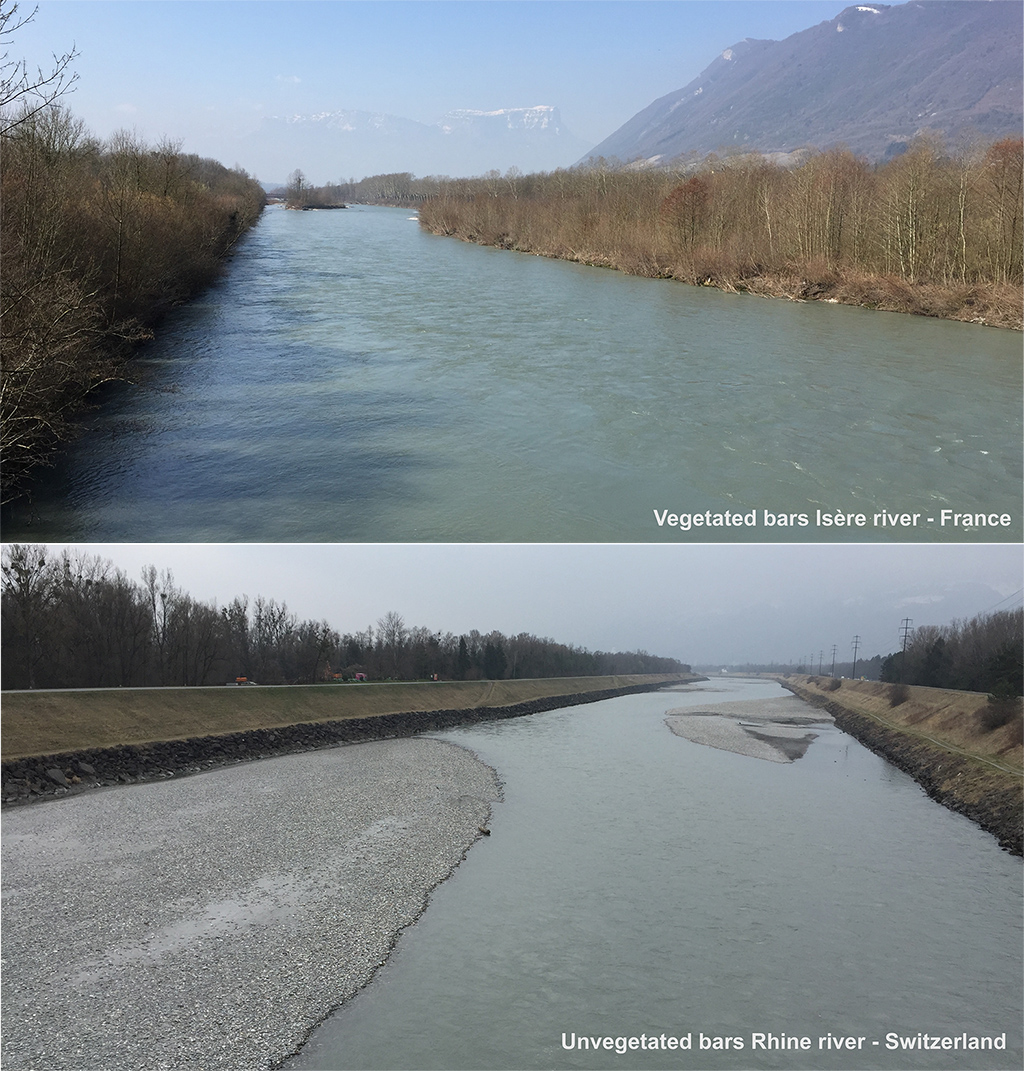
River managers and a society that aims at a sustainable interaction with the fluvial system are challenged by the need to ensure flood protection, water resources availability, and ecosystem health in a changing environment.
Understanding and predicting the interaction between riparian vegetation and river morphology is crucial to assess river evolutionary trajectories under different climate, flow regulation, and river restoration scenarios. In this context, the goal of BASEveg is to quantify important geomorphological processes that so far have been described only in qualitative way. This will be achieved developing a physically-based, quantitative tool integrating both eco-morphological and numerical expertise. Therefore, BASEveg aims to:
- advance and further develop the vegetation dynamic model to be included in existing two-dimensional models capable of predicting river morphodynamic changes;
- analyse the thresholds between vegetated and un-vegetated bed morphologies, as a function of flood intensity and the ratio between vegetation growth timescale and flood frequency, for different types of vegetation and different initial morphological configurations;
- investigate and modelling the morphological and vegetation dynamics and their interactions in a reach of the highly dynamic braided Tagliamento River in NE Italy, which is worldwide known as an reference river system for the interactions between flow, sediment transport and riparian vegetation dynamics.
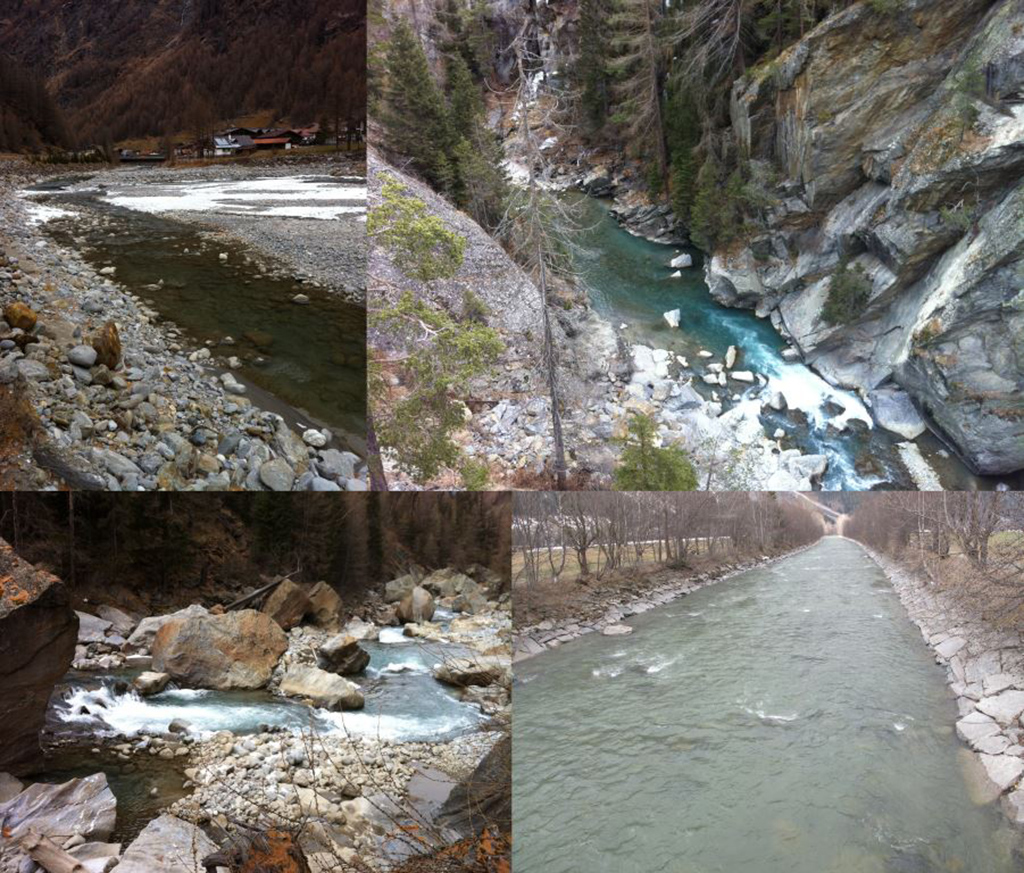
In order to increase the hydropower utilization, the hydropower company TIWAG is planning to access new catchment areas to contribute to the existing reservoir “Gepatsch” which belongs to the hydropower plant “Kaunertal” in Tirol. For this purpose, two new water intake facilities shall be built at the main tributaries of the “Ötztaler Ache”. To counteract reservoir sedimentation, an annual flushing of trapped sediments is being planned.
The long-term effects of sediment flushing are investigated by means of 1-D hydro- and morphodynamic simulations using the software BASEMENT. To meet the conditions of the study area, bedload transport was modelled using a multi-grain extension of the Smart & Jäggi transport formula.
Firstly, the actual state was modelled for the period 1997-2012. Sediments are being excavated along the “Ötztaler Ache” to some extend in the scope of flood protection. These removals play an important role and were implemented by different approaches in the numerical model.
Secondly, the sediment flushing during the operational phase of the intakes (planned state) was simulated for the same period of time. A comparison of the results of the actual and planned states was performed in order to evaluate the effect within the diverted river section downstream of the intake facilities.
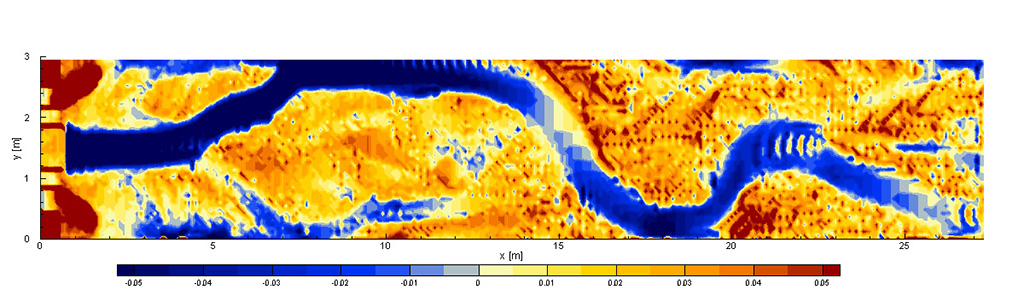
This computational study aims at describing and quantifying aggradation, degradation and channel rearrangement during floods by means of numerical modelling of braided rivers. For the validation of the numerical model, sophisticatedly monitored flume experiments provide topographical data as well as information on sediment transport with a high resolution in time and space.
The numerical model used to simulate temporal extracts of the flume experiments is based on the two-dimensional shallow-water equations solved with a finite-volume technique and explicit time discretization. Regarding sediment transport the numerical modelling approach includes diverse treatment of suspended load and bed load transport of sediment mixtures based on an active layer approach.
As far as sediment transport and according empirical relations are concerned, the focus is on bed load transport and the effect of bed armouring, which often occurs in alpine gravel-bed rivers. The numerical simulations are carried out with an initially braided topography based on an extract of the flume experiments and a constant hydrograph as steady inflow boundary condition. The results are verified with the data of temporally corresponding flume experiments. The impact of different bed load formulae and a varying resolution of the computational grid are studied. Furthermore the aspect of the different time scales of water and sediment transport is of interest.
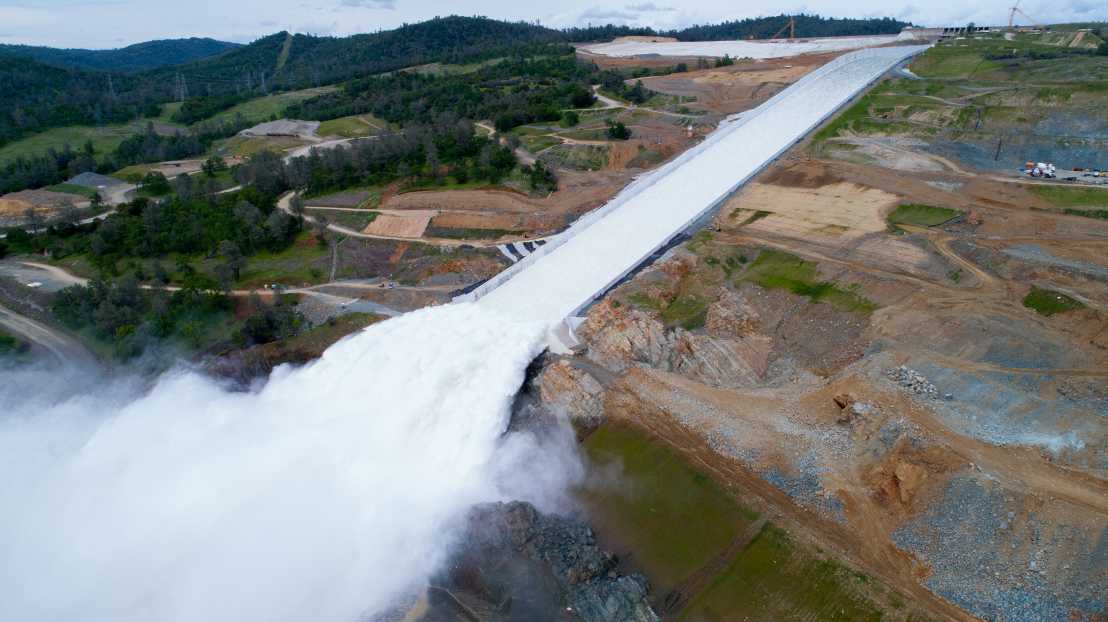
Reservoir dams play a crucial role for society and economy. Large outlet structures, such as spillways or low-level outlets typically ensure the dam safety in case of floods and convey extreme discharges. In the near future, spillways of many existing dams may need refurbishments due to aging infrastructure or to cope with larger flood magnitudes resulting from climate change. Therefore, there is a strong need for safe and reliable design guidelines.
High velocities and turbulent flow conditions typically result in self-aeration of the flow (Fig. 1). The accurate description of the resulting air concentrations along spillways are essential, e.g., to quantify flow bulking and the risk of overtopping of side walls, to quantify risk of cavitation along the spillway, or to design of downstream energy dissipation measures.
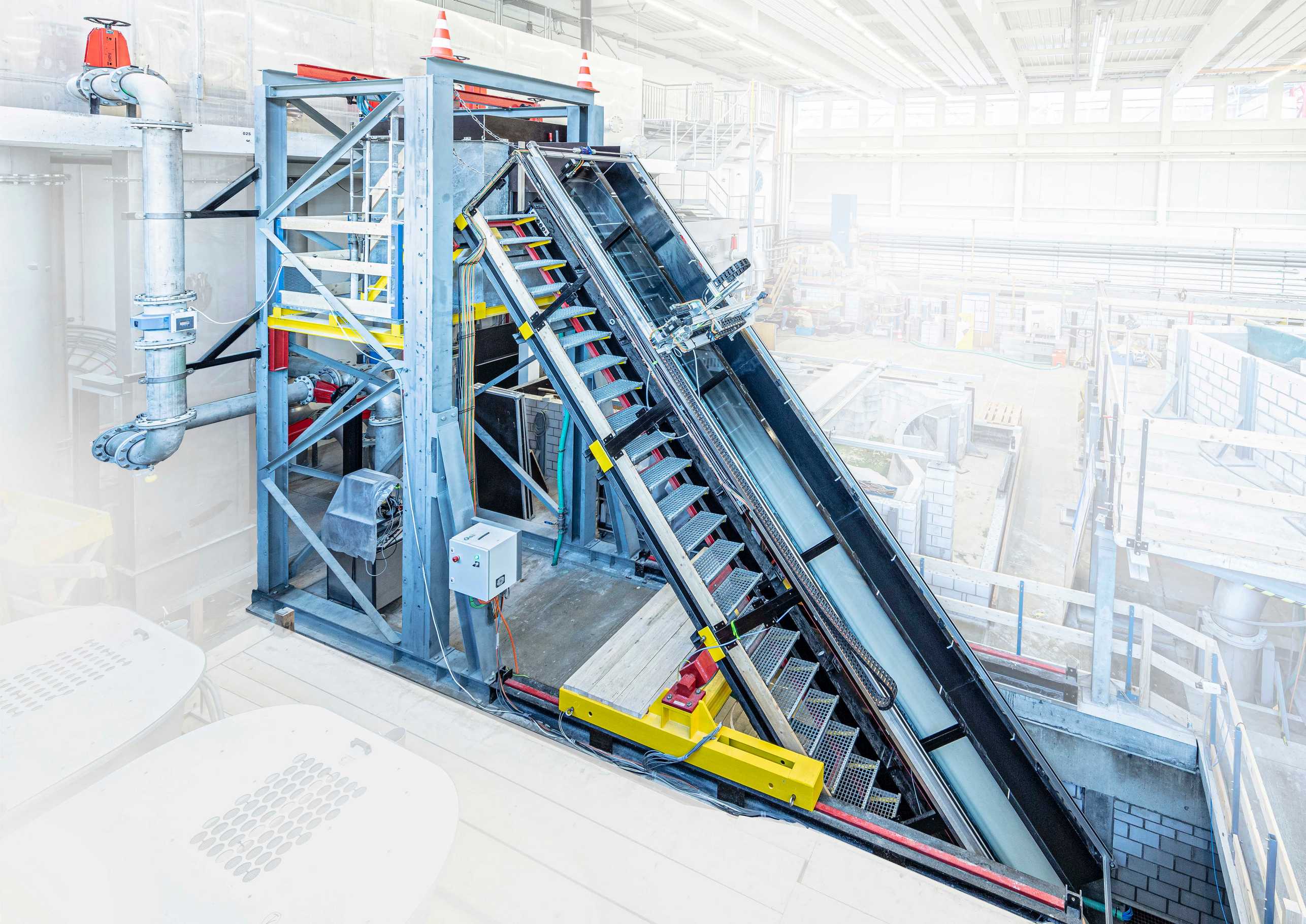
Most design recommendations are based on scale model tests which are often case-specific and may be subject to scale effects. Highly resolved numerical simulations are not yet feasible due to significant computational demands for flows in outlet structures. Thus, the prediction of air-water flow hydraulics along spillways remains challenging task.
The lack of a comprehensive data set on air-water flow properties in the developing aerated flow region is one the main identified knowledge gaps. Further, the lack of comprehensive dataset is a major reason for the limited quantitative description of the evolution of self-aerated flows on spillways.
The project “SAFAIR - Part B” targets the identified knowledge gaps. One of the main goals is to obtain a high-quality dataset from a large-scale hydraulic model of a spillway chute (Fig. 2). The model features a 9 m long and 0.5 m wide spillway chute. The experimental program consists of two different inclinations angles of 30° and 50°. To achieve different levels of turbulence, the invert roughness is varied by means of exchangeable plates with a homogenous washed concrete surface. The concrete plates are characterized by grains sizes of approximately 1 mm, 5 mm, and 10 mm, respectively.
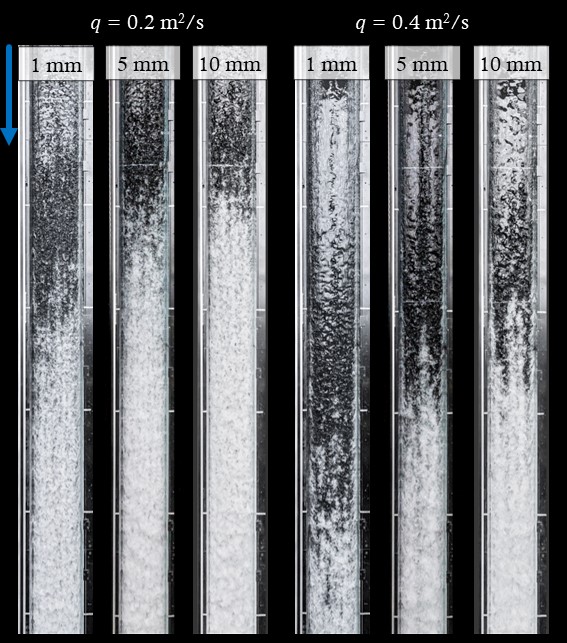
The measured and calculated flow properties include flow depths, flow velocities, turbulent stresses, air concentrations, as well as bubble/droplet frequencies and chord lengths. Further, inception point locations are evaluated based on visual observations (Fig. 3).
At the second stage of the project, a simplified numerical model for self-aerated flows on spillways will be developed and validated based on the dataset collected in the first part. This tool will be incorporated into the freely available BASEMENT software, thereby allow for safer and cost-efficient design of outlet structures.
A Description of SAFAIR – Part A is available here.
Within the next 30 years, many hydropower plants in Switzerland (collectively amounting to 23 TWh of electrical output) reach the end of their current concession. Therefore, the Swiss Federal Office for Energy (SFOE) in collaboration with nine project partners launched the HydroLEAP project to research different aspects of optimization of the environmental and operational costs for the modernization of the Swiss hydropower fleet.
In the scope of this project, the performance of the downstream fish protection and guidance measures are being assessed at the Massongex-Bex-Rhone (MBR) project site on the Rhone River where a new low-head hydropower plant (HPP) is planned with a design discharge of 220 m3/s (Figure 1). To facilitate the downstream fish movement, two different types of guidance structures are considered: a Horizontal Bar Rack – Bypass System and a Curved-Bar Rack – Bypass System.
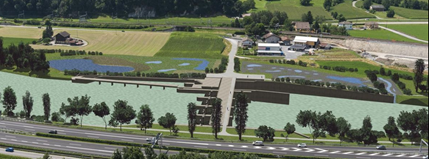
Computational fluid dynamics (CFD) is a powerful tool for investigation of flow under different operational or geometrical conditions, and a 3D model of the upstream part of the MBR HPP is set up in OpenFOAM (Figure 2) for the analysis of the following objectives:
- Flow conditions at the bar rack and optimal location of bar rack position
- Flow field downstream of the bar rack for different bar rack configurations and turbine admission flow
- Recommendations for optimisation of bypass design
Different geometrical variants of the head race channel and the positioning of the bed load diversion sill are considered to optimise the design of the fish guidance structure. The flow through the vertical Curved-Bar Rack and subsequent downstream flow field are further analysed by the use of a numerical detail model.
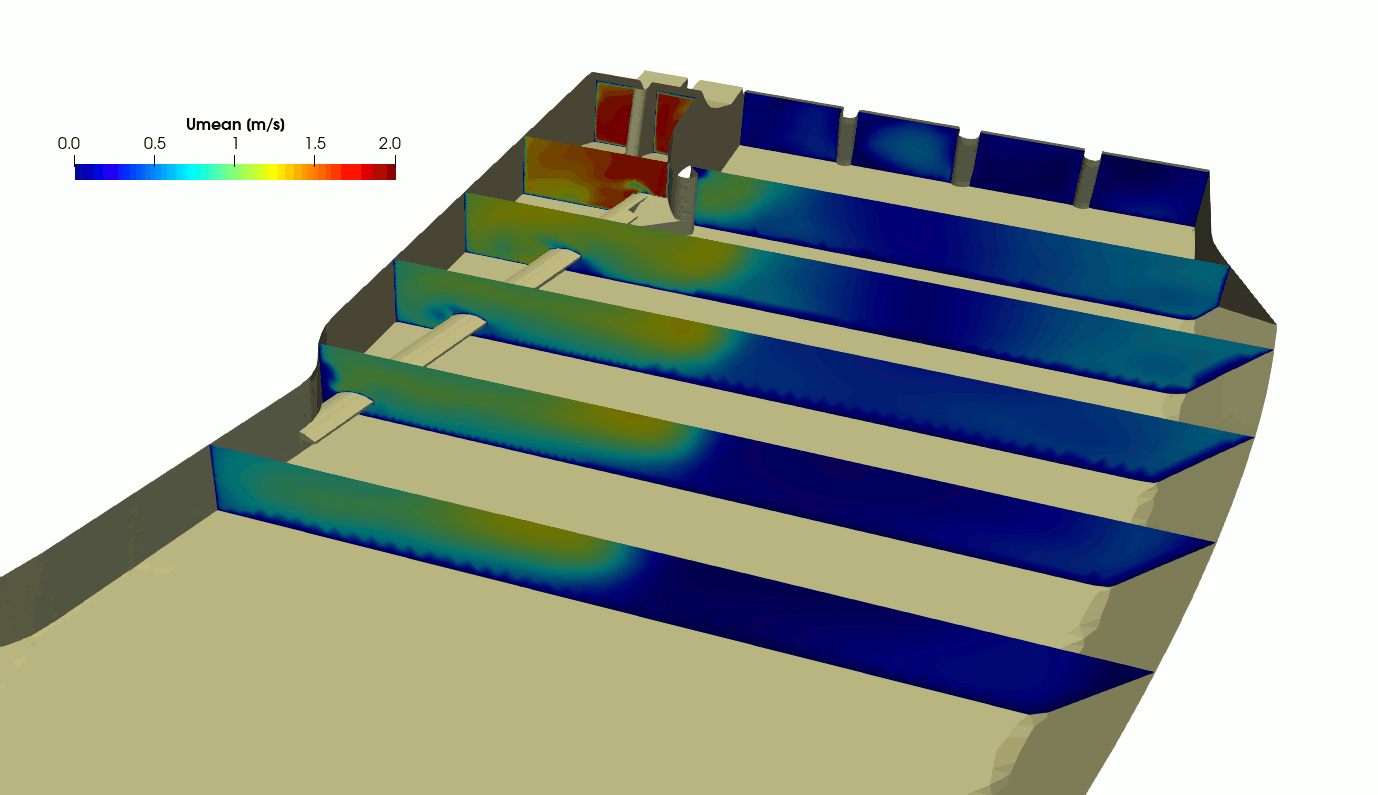
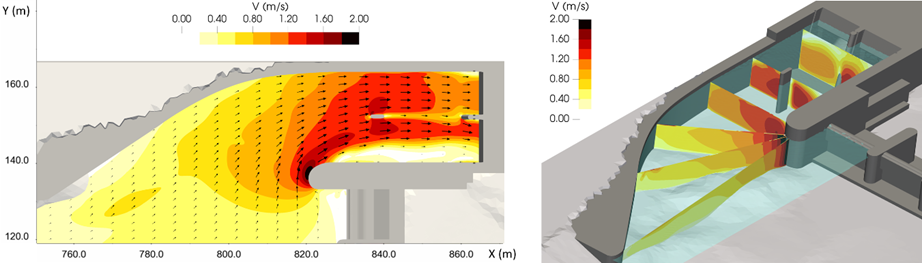
Man-made barriers like hydropower plants (HPP), weirs, and dams interrupt the longitudinal connectivity of rivers, which hinders migration of fishes and delimitates their natural habitats. The negative impacts on fish communities and consequently on the whole ecosystem underline the need to restore river connectivity allowing for up- and downstream migration of fishes again. While upstream fishways have been implemented successfully and are still being optimized, the focus of current research is more and more on fish guidance structures (FGS) for downstream migration. The proper positioning of FGS, as well as the optimization of upstream fishways, requires in depth knowledge of the site specific hydraulic conditions. Computational fluid dynamics (CFD) has evolved as a powerful tool for investigating the flow under varying operational conditions and therefore has a high potential for fishway designs, for both up- and downstream migration.
In the scope of this project the hydraulic conditions at three different power plants along the Aare River are investigated by means of numerical 3-D simulations using the software packages FLOW-3D and OpenFOAM. The 3-D geometries are constructed from bathymetry measurements of the adjacent river bed and construction plans of the HPP facilities.
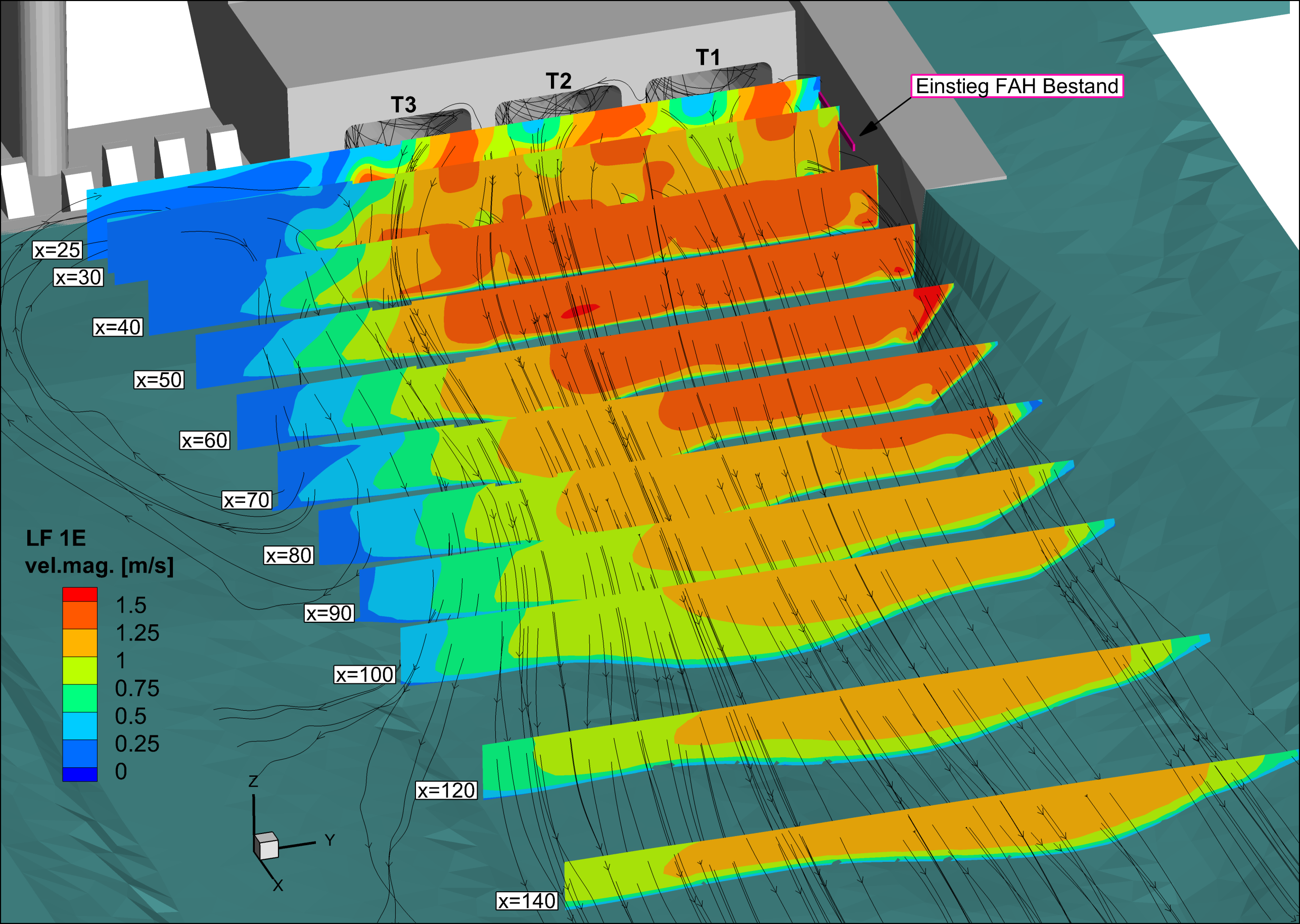
For the bay type HPP Brügg the upstream approach flow conditions are accessed for planning of future FGS in the headrace channel (Fig. 1).
Further downstream the Aare River between Solothurn and Olten at the block type HHP Bannwil, the focus is on the optimization of the existing upstream fishway. To this end, flow velocities downstream of the turbine outlets at the entrance of the fishway are of particular interest (Fig. 2). In order to account for the swirl effect of the rotating turbine runners, a specific boundary condition available in OpenFOAM is used to impose a radial velocity component.
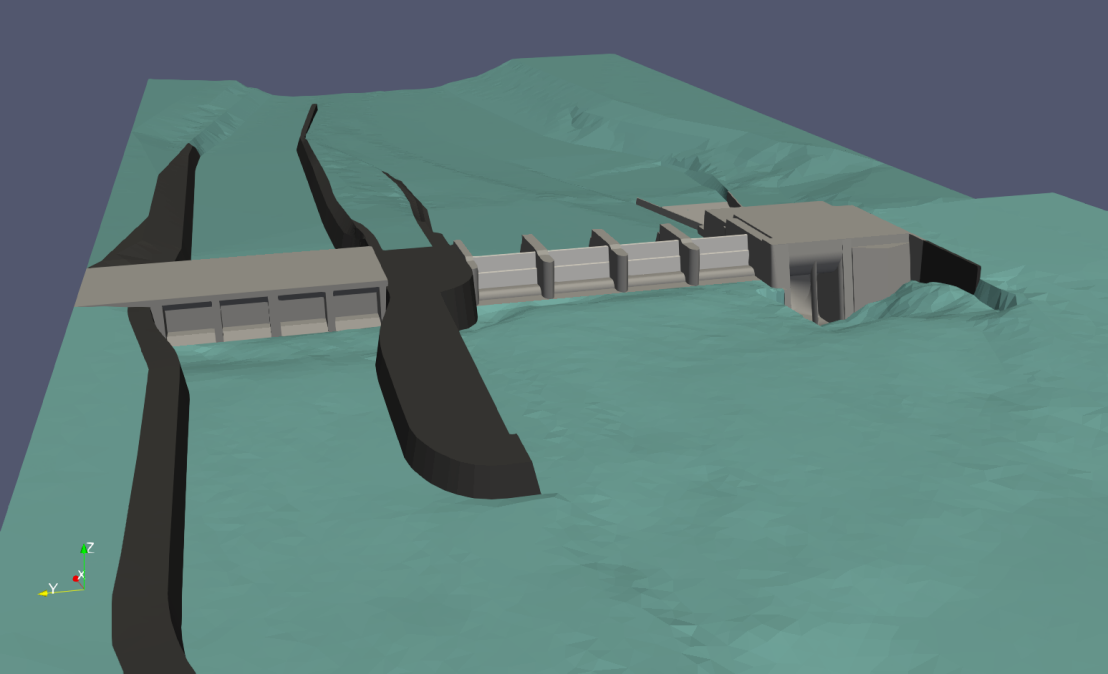
The facility Wynau/Schwarzhäusern, located about 5.5 km downstream of Bannwil, consists of a weir and two power houses (Fig. 3). First, simulations of the power plant approach flow conditions will be conducted to identify possible positions for future FGS. In order to validate the CFD results, ADCP measurements are conducted at all three locations.
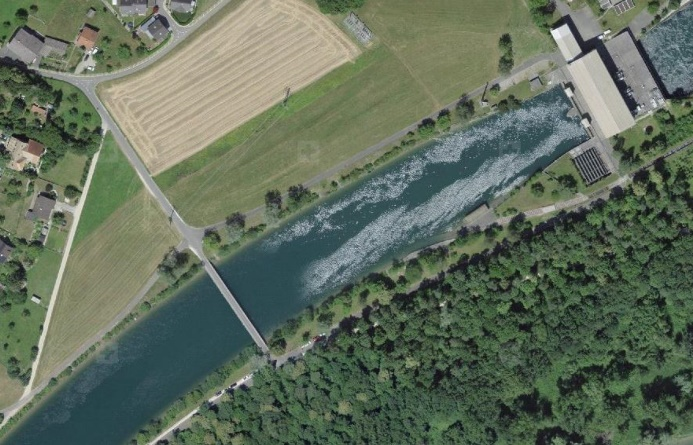
Run-off-river hydropower plants may be barriers to up- or downstream migrating fish. For this reason, efforts have been made to make hydropower plants passable for fish. While there are common measures for upstream migration such as fish ladders or bypasses, downstream migration of fish remains a challenge.
In 2011, the association of power plant operators at the Aare, Hochrhein, Limmat and Reuss rivers (VAR) launched a research project to establish and improve fish downstream migration at larger river power plants. In this scope, the feasibility of fish guidance structures with bypass at the power station Wildegg-Brugg will be investigated. The power station is equipped with two vertical Kaplan turbines, with maximum discharge of 420 m3/s in total. VAW is commissioned by Axpo Power AG to evaluate the optimal location and setup of a vertical bar rack for fish protection using a numerical 3D model. The following main issues are investigated:
- flow field in upstream channel and turbine approach flow
- optimal location of vertical bar rack upstream of power station
- head loss of different vertical bar rack configurations
- effect of vertical bar rack configurations on turbine approach flow
The head loss induced by the vertical bar rack is assessed by the use of a numerical detail model of the rack. Different configurations and bar shapes are being tested in order to optimize hydraulic loss at the rack and subsequent turbine admission flow.
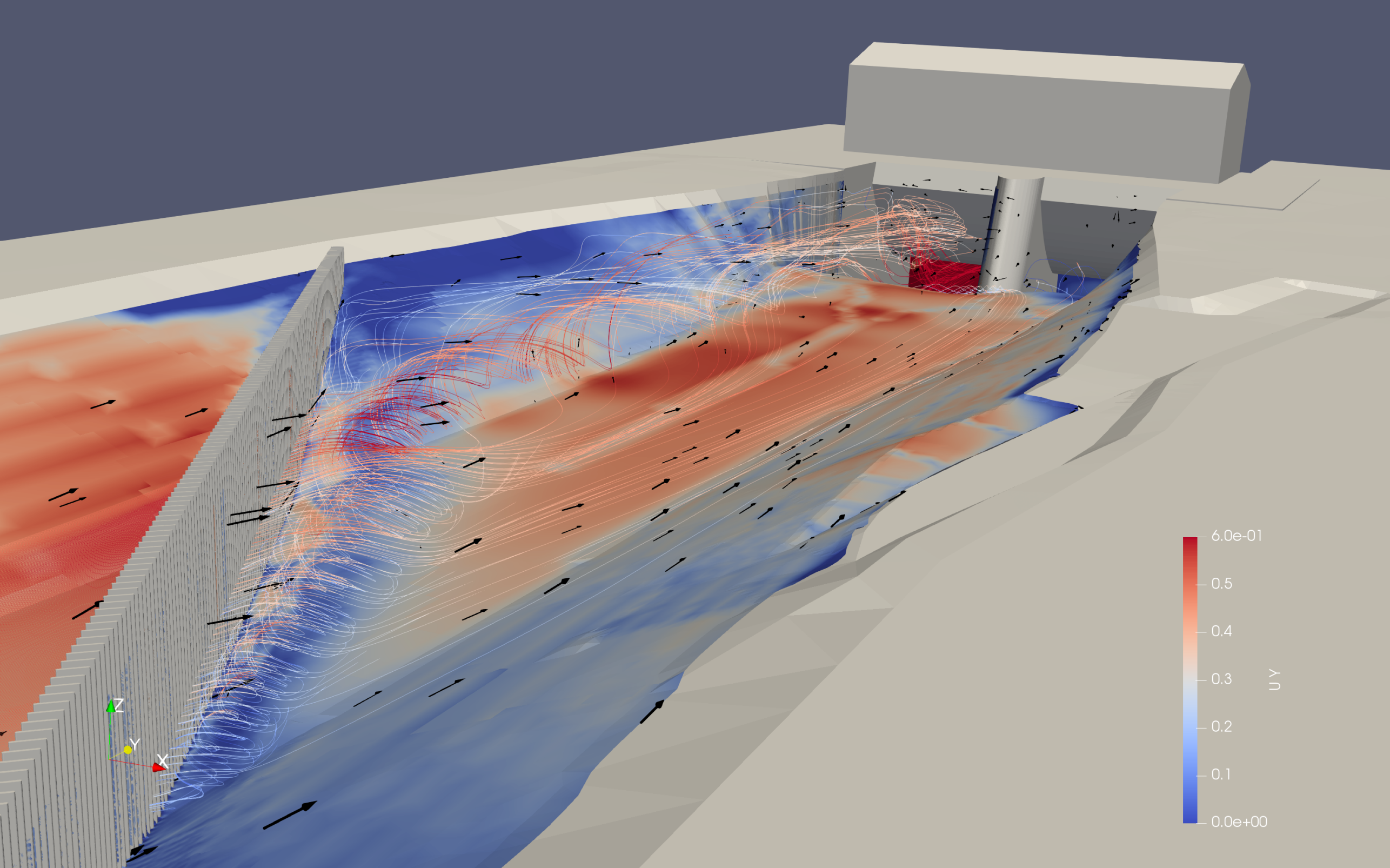
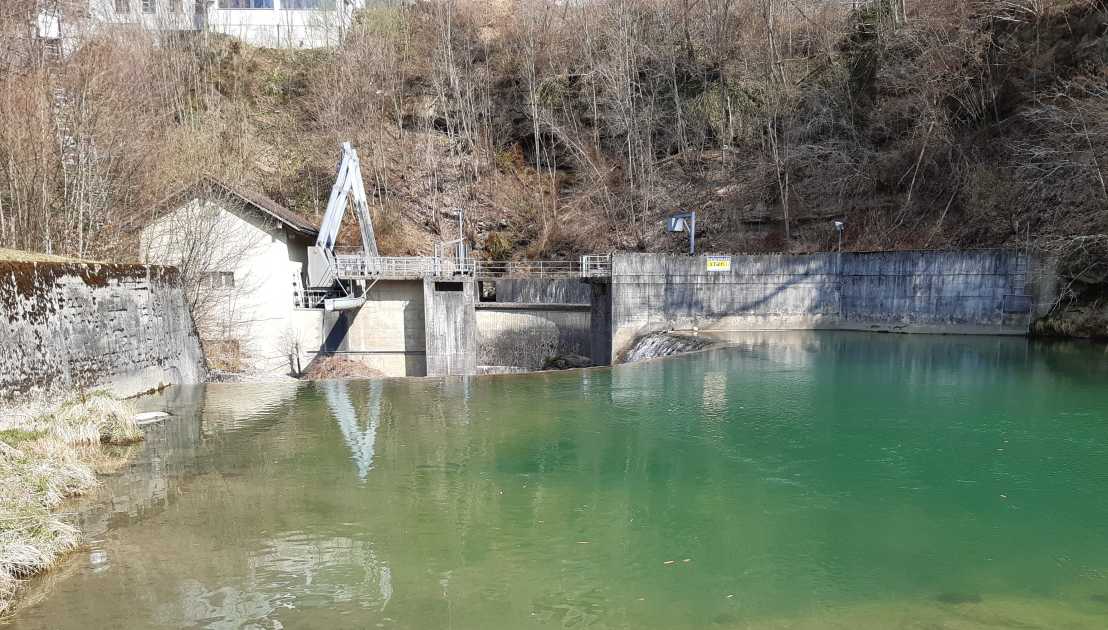
The fish downstream migration is hindered at many hydropower plants in Switzerland. The operator St.Gallisch-Appenzellische Kraftwerke AG (SAK) is developping a pilot project at the river Thur in the Canton St.Gallen in Switzerland to improve fish migration. The design discharge at HPP Herrentöbeli amounts to 11 m3/s and the HPP is equipped with two Kaplan turbines. In the current state, there is no residual flow unless the discharge of the Thur is above the design discharge (weir overflow). Therefore, fish downstream migration is only possible through the turbines with a high risk of injuries or mortality due to blade strike and strong pressure gradients.
The mitigation project will include the application of a Curved-Bar Rack (CBR) at the hydropower plant Herrentöbeli, which will guide fish to a new bypass through which they can safely migrate downstream. Detailed investigations will be conducted to gain further knowledge about the applicability of CBRs for fish downstream migration at other locations. A modified bar shape was specifically developed to minimize clogging.
Before installing the CBR in the field, laboratory experiments with live fish will be conducted to evaluate the performance of the CBR in the numerically determined setup. The fish guidance and protection efficiency will be analyzed during live fish tests using wild brown trout. Further investigations include the behavior of the CBR in relation to driftwood and the validation of the numerical results with respect to hydraulic head losses.
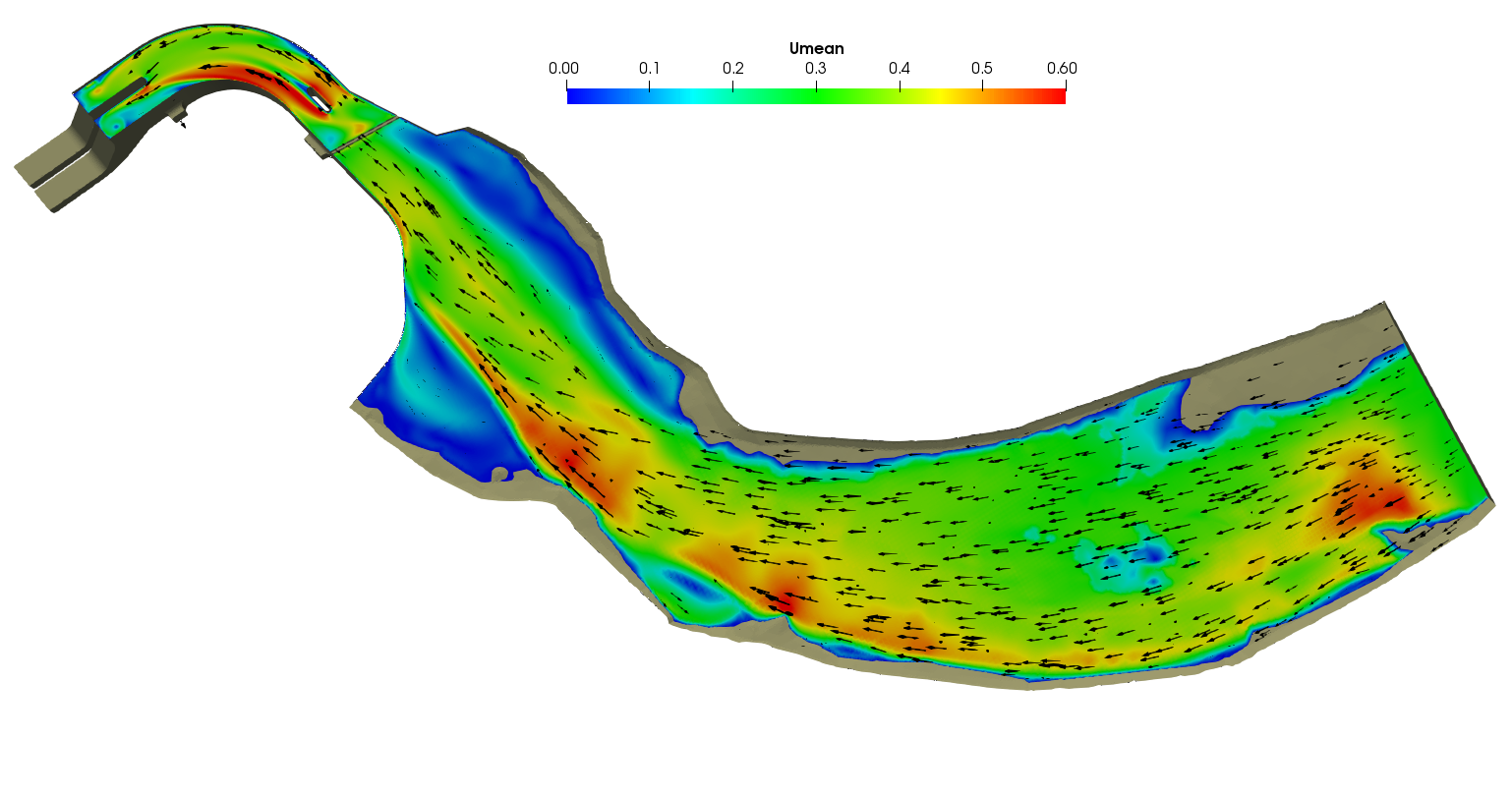
VAW was commissioned with a detailed analysis of the local conditions by measuring the topography as well as the flow field upstream of the HPP using ADCP-measurements. With the results a 3D model is set up, which is used for numerical simulations to reproduce the flow conditions in the forebay and in the admission flow channel of the HPP. The outcome of the simulations will allow to evaluate different CBR positions. In a further step, a detailed model is used to compute the hydraulic losses and to evaluate the flow field in the close vicinity of the CBR to assess its fish guidance capacity.
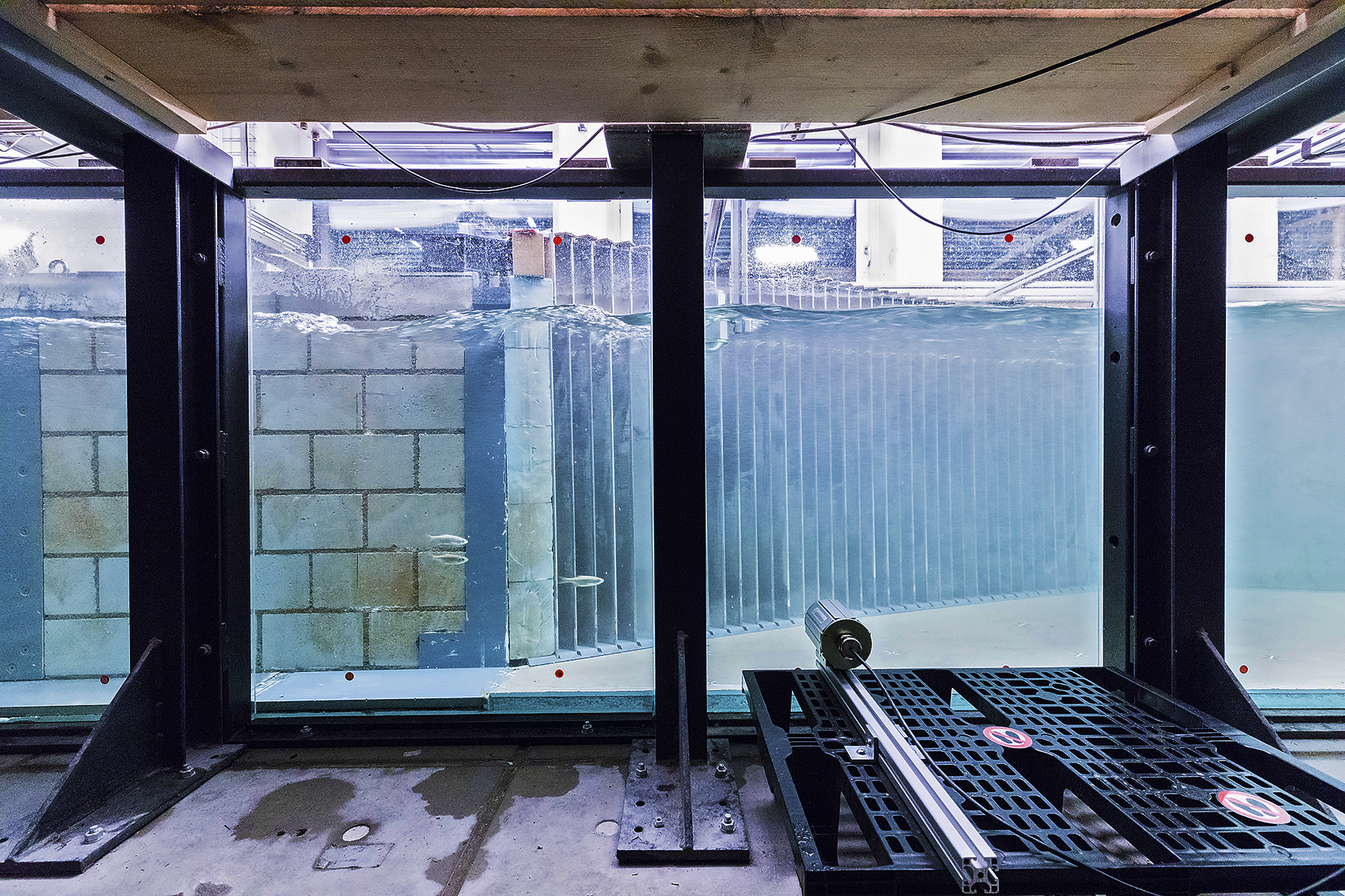
![Enlarged view: Proof of Concept of the Workflow: Case Study on Lake Lucerne. Wave generation, propagation and run-up via a trigger given by two simultaneous submerged landslides. [Animation: P. Bacigaluppi, © 2017 swisstopo (JD100042)]](/en/research/cfmd/research-projects/_jcr_content/par/textimage_1803793676/image.imageformat.text50percent.747426394.gif)
Tsunamis in lakes represent one of the natural hazards in Switzerland. On a historical basis, inland lakes have been subject to severe wave inundations in the past, with major events as Lake Geneva in 563 AD and Lake Lucerne in 1601 AD, with reported wave heights of several meters and high destructive impact on adjacent settlements on the shoreline. The trigger mechanisms, preconditions, processes and impacts of lake tsunamis are currently still under-explored and therefore no warning mechanisms are yet available. This project is within the interdisciplinary SNSF Sinergia research project.
The objective of the numerical modeling is to provide a workflow for the probabilistic hazard assessment of tsunami events on Swiss lakes. In particular, the wave generation, propagation and run-up of tsunami waves will be modeled, to allow a future estimation of the inundated area and flood propagation velocities. There are two main investigation strategies: i) numerical simulations carried out via BASEMENT v3 will be integrated actively within the workflow of the Sinergia project; ii) laboratory experiments will be used to verify numerical evaluations.
The outcome of this challenging project will help to enhance the understanding of the process and will substantially contribute for assessing the risks for people, lifelines and structures along lake-shores and coasts. This study will contribute in defining the needs and benefits of potential mitigation strategies.
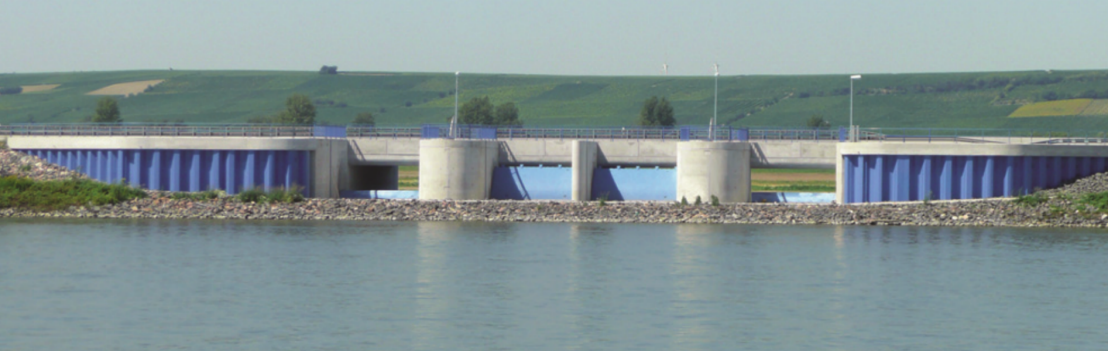
Flood discharges may lead to inundation that threatens people and buildings as well as damages agricultural land. Flood polders are a measure, particularly common in Germany, to reduce flood peaks and thus mitigate flood disasters. A flood polder is a retention area arranged laterally to the river, where a large volume of water can be stored during a flood event.
The inlet structure of a flood polder is an important component of a well-functioning flood retention system. Inlet structures are used to divert the discharge when the flood level rises and thus to reduce the flood peak discharge in the river. Inlet structures can be distinguished according to their controllability. Controlled inlet structures are classically equipped with flaps or gates. Uncontrolled structures may be designed as overflow dams or are equipped with tilting elements, for instance.
In order to support the design of flood polder inlet structures, this project compiles various possibilities of types and arrangements of inlet structures. For this, a literature study is carried out. In addition, a survey addressed to operators of flood polders is launched to collect data and experience about the construction, operation and maintenance of inlet structures.
The different types of inlet structures are compared and evaluated using criteria such as costs for construction and maintenance, robustness, flexibility with regard to flood management, impact on the landscape, etc. For the implementation of the inlet structure, the environment and boundary conditions (e.g. risk of drift wood clogging, accessibility of the structure, etc.) are essential and must be taken into account for the evaluation.
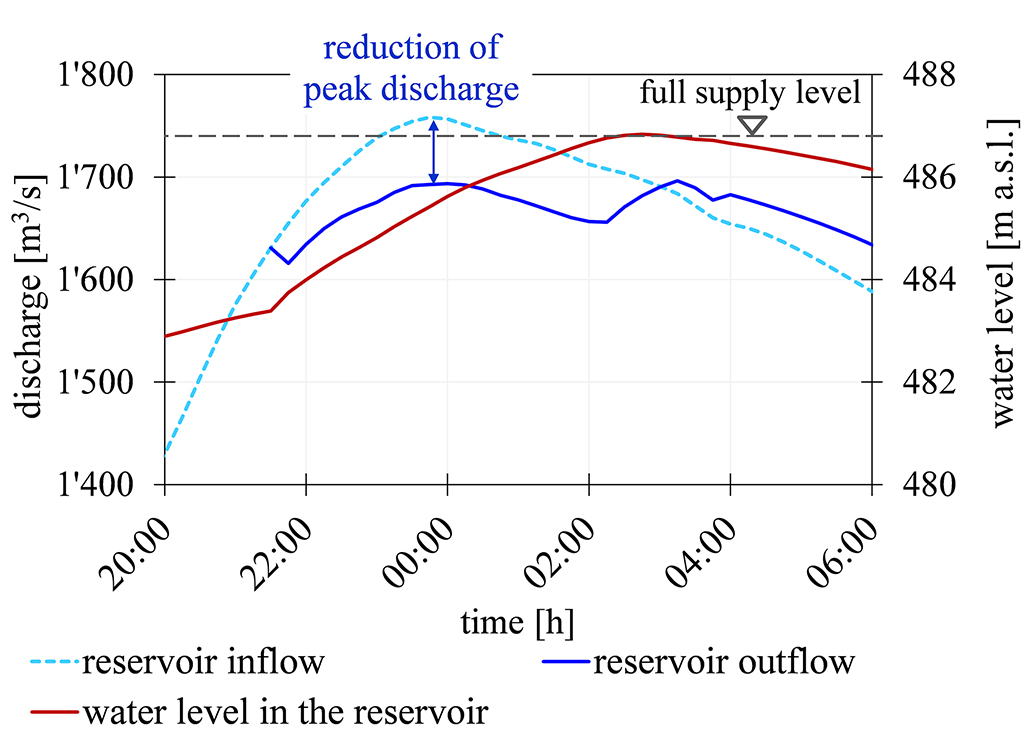
The construction of a run-of-river power plant may involve the separation of floodplains from the river. Consequently, the flood peak discharge will not be reduced due to inundation of the floodplains anymore and thus the flood wave will not be damped by retention. This may lead to more acute flooding problems downstream of the power plant, especially for people and buildings in densely populated regions.
The run-of-river power plant Langkampfen on the Tyrolean Inn, operated by TIWAG – Tiroler Wasserkraft AG, was built in 1998. Due to the construction of the weir and the embankments along the river, a reservoir has been formed. This artificial reservoir shall be used to reduce the flood peak discharge. The objective is to control the weir during the passage of the peak discharge to refill the storage and to facilitate an optimal capping.
The volume of the instream reservoir is small relative to the flood volume of the Inn river, so that only peak discharge capping becomes possible. The potential retention volume depends on the discharge as well as the corresponding water level. The higher the discharge, the lower is the retention volume. Furthermore, a forecast of the flood hydrograph to be capped must be provided for optimal retention.
The flood routing and the control of the weir are simulated with a hydrodynamic 1-D model developed at VAW, namely BASEMENT. The model includes a PID (proportional integral derivative) controller that can be used for controlling the water level in the reservoir. The objective is to adapt the PID controller automatically to the situation before, during and after the flood event. Therefore, requirements for the controller regarding the gate operation during the passage of the flood wave must be predefined. For the capping operation, the main constraints are not to exceed the normal operation water level and to comply with the freeboard regulations. The model is tested by investigating past flood events and possible scenarios. Finally, the model will be implemented for real time operation to provide recommendation to the operator for weir control during a flood event.