Research projects
High-speed flows
Hydraulic structures
- Koysha HEP – Physical model investigation of the gated spillway
- Assessment of the remaining useful life of Pelton turbines
Hydroabrasion and sediment monitoring
- Controlled fine sediment venting via waterways of storage hydropower plants with Francis turbines as a strategy to counter reservoir sedimentation (SedVent)
- Hydro-abrasion at hydraulic structures and steep bedrock rivers
- Field investigation on suspended sediment, hydro-abrasive erosion and efficiency changes of coated Pelton turbines in the high-head hydropower plant Fieschertal
- Field monitoring of sediment transport, hydraulics and hydro-abrasion at Swiss Sediment Bypass Tunnels
- Optimization of high-head small hydropower plants at sediment-rich rivers (Case study on sand trap efficiency and turbine erosion at the SHPPSusasca)
Reservoir Sedimentation
Ethohydraulics
- Movement behaviour of Brown trout parr during simulated hydropeaking
- Electrified fish protection structures for safe downstream fish passage at water intakes
- Advanced Bypass System for Downstream Migration of European Key Umbrella Fish Species (ABSYS)
- Turbulent eddies to create paths for safe downstream migration for salmonids and eel (FishPath)
- Fish downstream passage over weirs at low-head hydropower plants: Field study of total dissolved gas
- Development and application of an automated 2D fish-tracking software (Part I and II)
- Fishfriendly Innovative Technologies for hydropower (FIThydro) - Swiss case studies
- Measures to facilitate safe downstream fish migration at run-of-river hydropower plants
Dike breaches
- Composite Modelling of Dam Breaching due to Overtopping
- Internal erosion detection in dikes and earthen embankments using distributed fiber optics (DFO) technology
Impulse waves
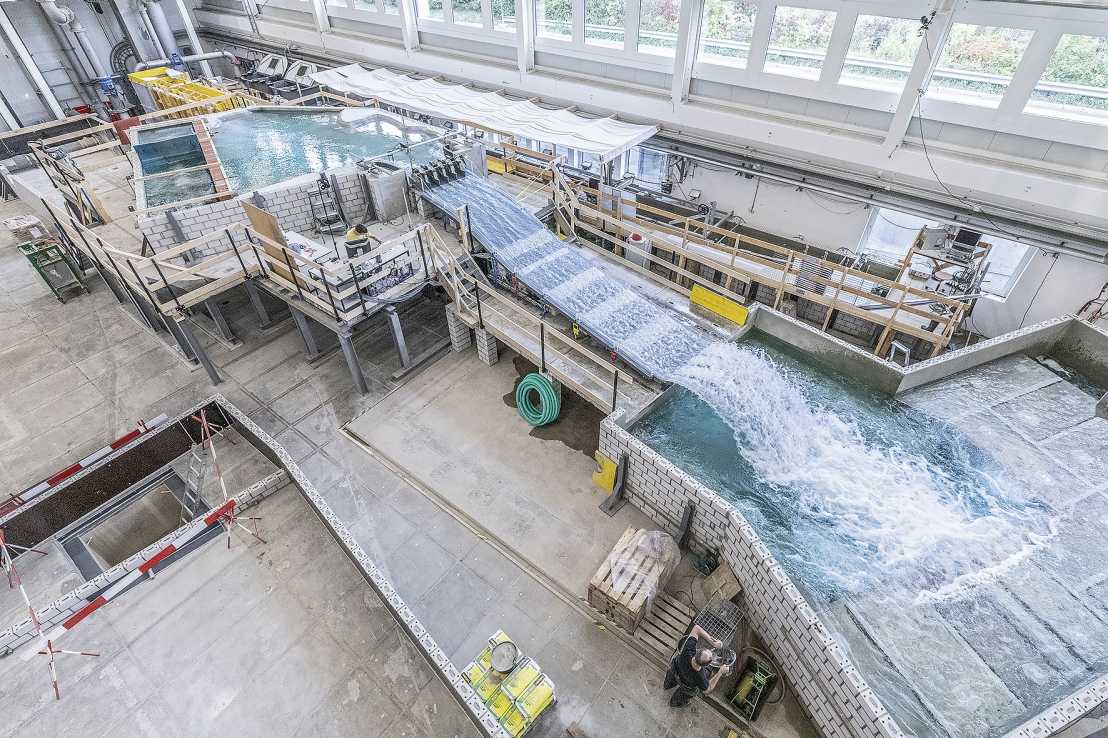
The Koysha Hydro Electric Project is located at the Omo River in southern Ethiopia. The plant has an installed capacity of 2’200 MW and will produce 6’500 GWh of electricity per year. Owner and project execution institution is the Ethiopian Electric Power Corporation (EEPCo).
The 178 m high roller compacted concrete (RCC) gravity dam impounds a storage volume of 6’000 million cubic meters. The dam is crossed by three power waterways feeding an outdoor power house which is equipped with eight turbines located at the left riverside bank (Figure 1). Two Download middle level outlets (PDF, 1.2 MB) are foreseen to be located in the central part of the dam. A gated spillway is a separate and independent structure on the left bank with the purpose of discharging water back into the Omo River downstream of the dam and the powerhouse.
The gated spillway consists of an approach channel leading to the control structure which is divided into six bays. Each bay is controlled by a tainter gate. The control structure is followed by a 580 m long and 120 m wide rectangular chute with a flip-bucket at its end. A plunge-pool downstream of the spillway will dissipate the energy from the spillway jet. Design discharge of the control structure and the spillway chute is equal to the routed 10’000-year flood of 13’150 m3/s. The design head HD for the spillway crest is 16.8 m, corresponding to the 5’000-year flood of 12’400 m3/s.
VAW is commissioned by the contractor Salini Impregilo S.p.A. to perform two physical model investigations on the spillway at a scale of 1:50 and 1:80. The partial model at a scale of 1:50 includes a section of four control structure bays and a part of the approach channel and the chute. The complete model at a scale of 1:80 includes all six bays of the control structure, a part of the approach channel, the spillway chute and its flip bucket, the plunge pool basin and a part of the Omo River.
The main purpose of the investigations is the verification and optimization of the ogee crest, the piers and the inlet side walls of the control structure, the optimal position and layout of the aerators embedded into the spillway, the pressure head distribution along the flip bucket, the trajectories of the outgoing jets and the performance of the plunge pool basin in order to guarantee their hydraulic performance.
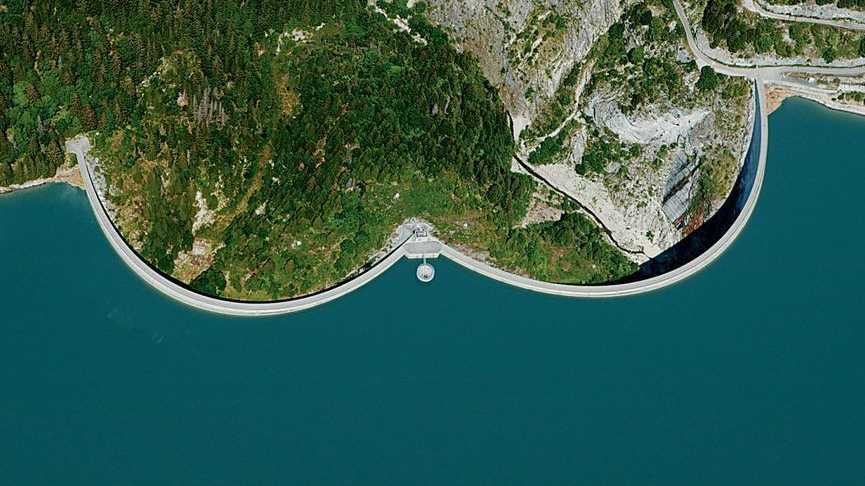
In the context of the Energy Strategy 2050, Swiss hydropower (HP) operators and other HP players are facing new challenges in terms of power plant operation and maintenance. Due to the massive development of new renewable energies, more and more facilities will be operated in harsh conditions to maintain grid stability. The number of starts and stops undergone by turbines has already risen and will further rise considerably, leading to a faster deterioration and fatigue of the turbine components. In addition, the turbines are operated in a wider load range than previously, leading to larger mechanical solicitations.
In Switzerland, Pelton turbines account for 38% of the hydropower generation. Their peak efficiency exceeds 90%, making it one of the most efficient turbine types. Pelton turbines are subjected to high hydro-mechanical forces, due to the high-speed water jets that impinge the buckets of the runner. These cyclic stresses generate fatigue cracks that might remain unnoticed until a machine inspection, which is time-consuming and costly because the turbine has to stand still during the whole inspection procedure. In the worst case, these fatigue cracks could propagate rapidly and lead to the complete failure of the bucket. Therefore, developing new diagnosis tools are crucial for the assessment of the so-called Remaining Useful Life (RUL) of the machine. Understanding how these different types of damages influence the dynamic response of the machine is thus of primary importance in the context of predictive maintenance, putting the power plant operators in good conditions to take appropriate decisions and reduce maintenance costs.
The project aims at deepening the understanding of typical Pelton ageing damages and their impact on the dynamic behaviour of the runner and the overall machine. The project is performed in the framework of the SFOE P+D project HydroLEAP, and more precisely on the FMHL demonstrator in Veytaux (Canton of Vaud). The focus is put on horizontal axis Pelton configurations at prototype scale.
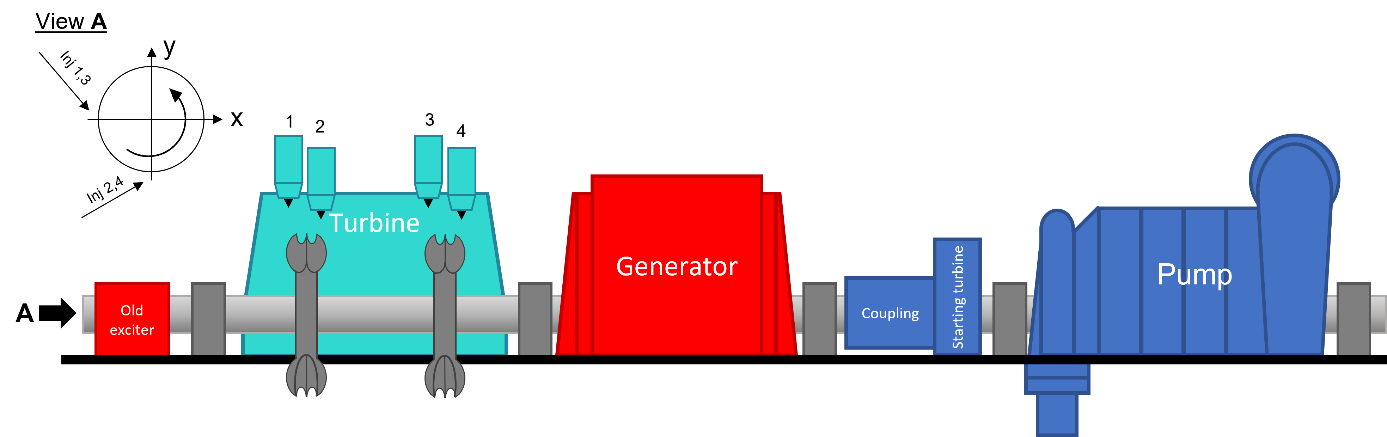
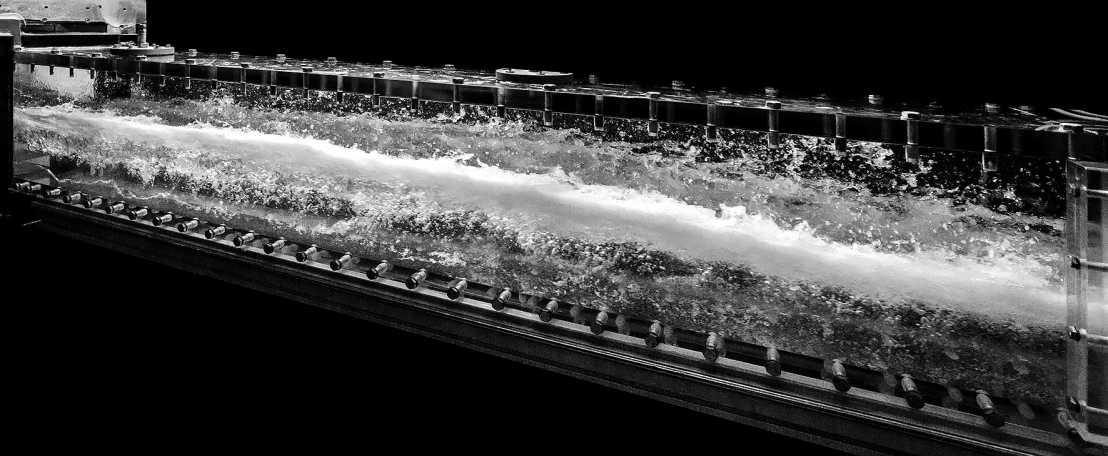
Reservoir dams play an important role in modern society as they serve many purposes such as hydropower energy production, sediment flushing, water supply, flood control, irrigation and, to a smaller extent, recreation, navigation, and fish farming. Reservoir dams feature large outlet structures such as spillways and low-level outlets. The latter are key safety devices which allow for a fast drawdown of the reservoir in case of maintenance and emergency situations, or sediment flushing and environmental flow release during normal operation. In low-level outlet tunnels, high velocities and turbulence levels of the free-surface flow cause air entrainment and air transport along the tunnel, resulting in sub-atmospheric air pressures. This may lead to cavitation, gate vibration and slug flow, endangering the operational and structural safety of the outlet. These problems can be mitigated by proper aeration via an adequately designed air vent.
The overarching goal of this research is to develop a physically based method that enables estimating the evolution of air-water flows, incorporating knowledge of large-scale physical modelling and novel instrumentation techniques (i.e., multi-tip phase-detection probes), to allow for a safe and cost-efficient design of future outlet structures in dams as well as to reliably reassess the hydraulic safety of existing dams for a changing climate. The project is divided into Part A and Part B, carried out by Simone Pagliara and Matthias Bürgler, respectively. In Part A, detailed physical model tests will be conducted focusing on low-level outlet flows and their upscaling to prototype. A description of part B is available here. The main goals of part A are: (i) to validate the multi-tip phase-detection probe in a custom-made test rig; (ii) to quantify the scale effects affecting the air demand estimation in low-level outlets and to investigate the effects of (iii) tunnel profile transitions, (iv) tunnel roughness, (v) bottom and sidewall aerator on air demand and low-level outlet performance.
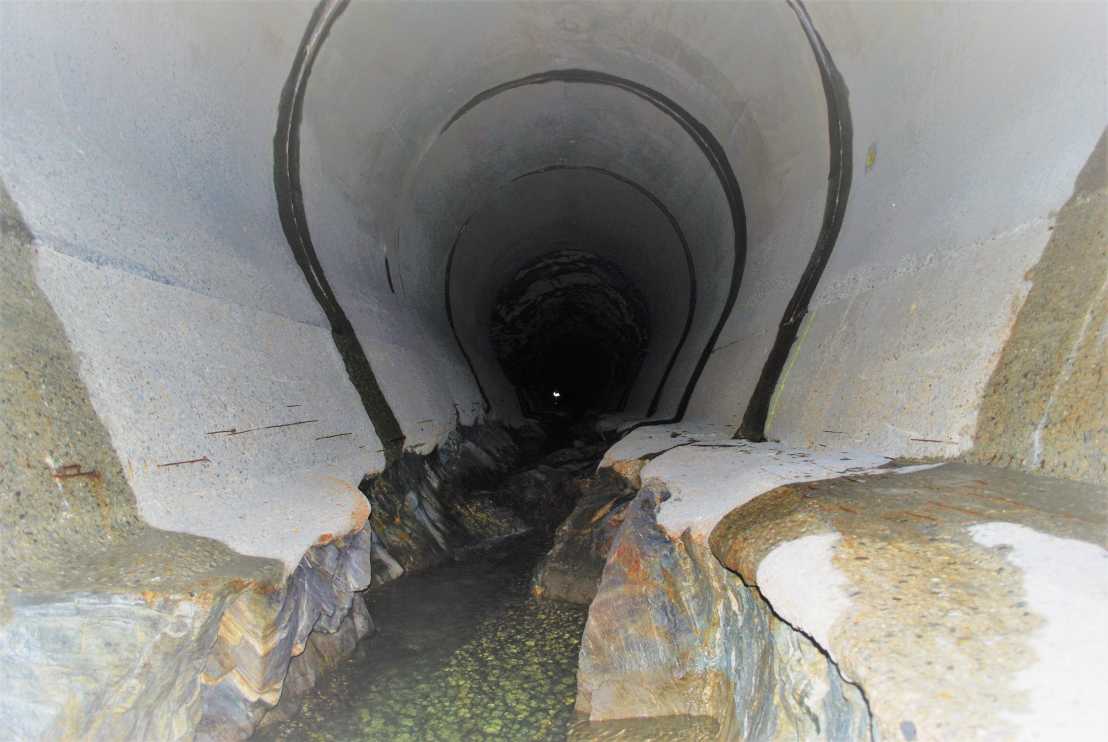
The sustainability of many reservoirs is threatened by sedimentation. The need for active sediment handling using sediment bypass tunnels (SBT), or sediment flushing and sluicing facilities (SFSF), amongst others, will consequently increase considerably in the future to maintain reservoir sustainability as well as sediment connectivity. However, high speed flows and high sediment transport rates can cause severe hydro-abrasion at such hydraulic structures as well as river bed incision. Better understanding of the abrasion mechanics and development of a realistic abrasion model are keys for both design and sustainable use of hydraulic structures, and for landscape evolution modelling.
The project aims at improving knowledge on the physical processes of turbulent flow characteristics, bedload particle motion, and hydro-abrasion and their interrelations in high speed flows. Effects of flow velocity, water depth, particle size, hardness, shape and transport rate on hydro-abrasion of various bed materials will be systematically investigated in a laboratory flume, simulating SBT, SFSF and high-gradient mountain streams. In addition, field data from existing SBT in Switzerland and literature data will be collected to use upscaling the laboratory results to prototype dimensions. Finally, an abrasion model to forecast hydro-abrasion at both laboratory and prototype scales will be developed.
The project is of prime importance for hydropower companies, hydraulic engineers, and federal agencies dealing with energy (Swiss Federal Office for Energy), water supply and environment (Swiss Federal Office for the Environment) as well as for the research community. Furthermore, the project contributes to a successful realization of the ‘Energy Strategy 2050’ of Switzerland.
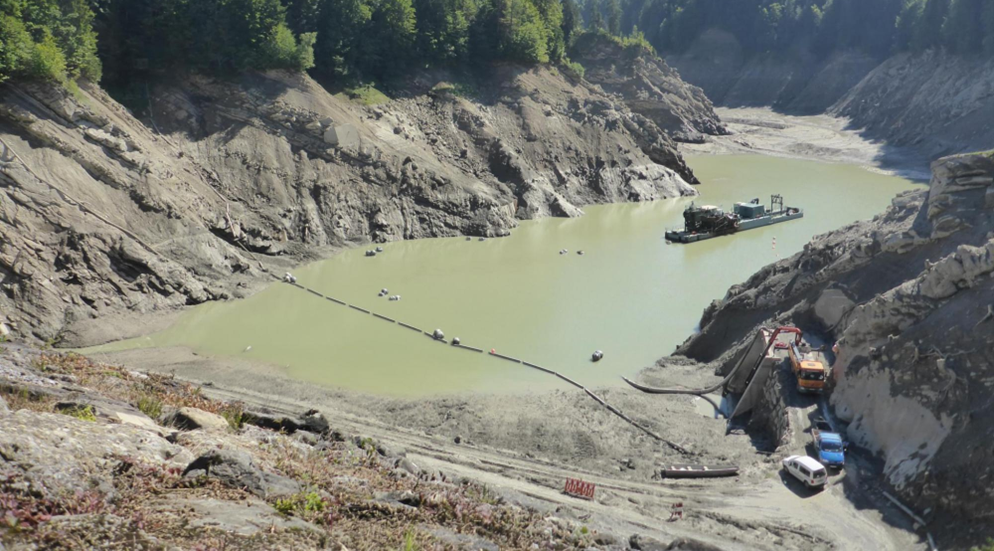
For decades, storage hydropower has been of great importance for the electricity supply in Alpine and adjacent regions. On one side, it generates electric energy, and on the other side, reservoirs allow to store energy. Given the increasing risk of winter supply shortages and the requirements of the future energy system, there is a high necessity of maintaining and extending hydropower capacities. However, large amounts of sediments are transported into hydropower reservoirs and deposited there every year (Fig. 1). The sedimentation leads to a loss of storage capacity and compromises the functionality and operational safety of hydropower intakes and outlet works.
One strategy to counter reservoir sedimentation is the so far rarely applied systematic venting of fine sediments via the power waterway of hydropower plants. Thereby, production losses and high suspended sediment concentrations, as typically occur during flushing, are avoided. However, the increased fine sediment load poses a challenge in terms of hydro-abrasive erosion on the turbines, reducing their efficiency and leading to lower electricity production. The costs of fine sediment venting depend, amongst others, on the maximum particle size and the maintenance strategy. These topics are addressed in a case study at the Bolgenach reservoir and the connected hydropower plant Langenegg in Vorarlberg, Austria.
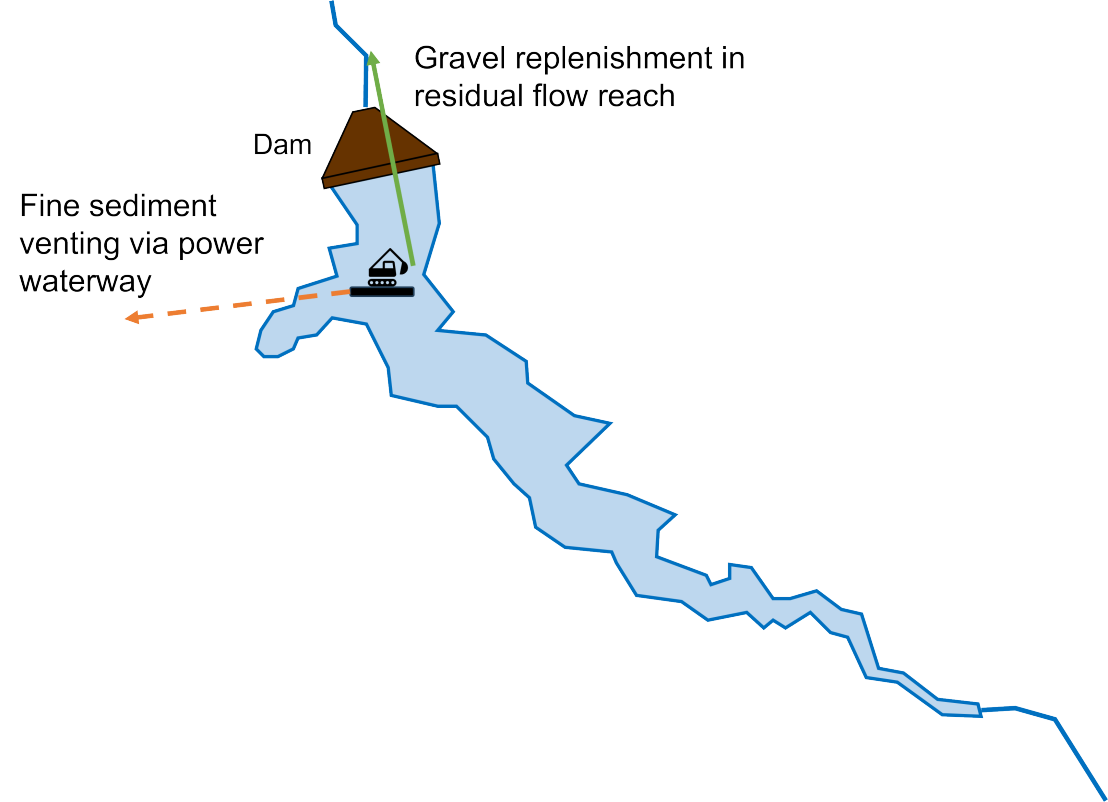
As part of the new sediment management system (Fig. 2), deposited sediments in the Bolgenach reservoir are dredged and sorted by size on board a vessel. Fine sediments are pumped to the intake and vented through the power waterway, through which they are delivered to the river reach downstream of the powerhouse. Coarse particles are transported to the bottom of the dam by a cable car, where they serve for sediment replenishment in the residual flow reach and thus for ecological improvement.
The fine sediment load of the turbines is quantified by measuring particle concentrations in real-time, in addition to particle properties like size, hardness, and shape. In parallel, the hydro-abrasive erosion at the Francis turbines of the hydropower plant and their efficiency are monitored. The study aims to optimize the venting process with respect to technical and economic aspects while complying with environmental regulations. In addition, the turbine erosion model according to IEC 62346 shall be further calibrated and validated. Using this model and based on the operation experience, the management and maintenance strategy will be improved.
Additional knowledge on fine sediment venting via power waterways of hydropower reservoirs contributes to the sustainable use of the hydropower potential and allows to better cope with sediment loads at reservoirs in the future considering climate change.
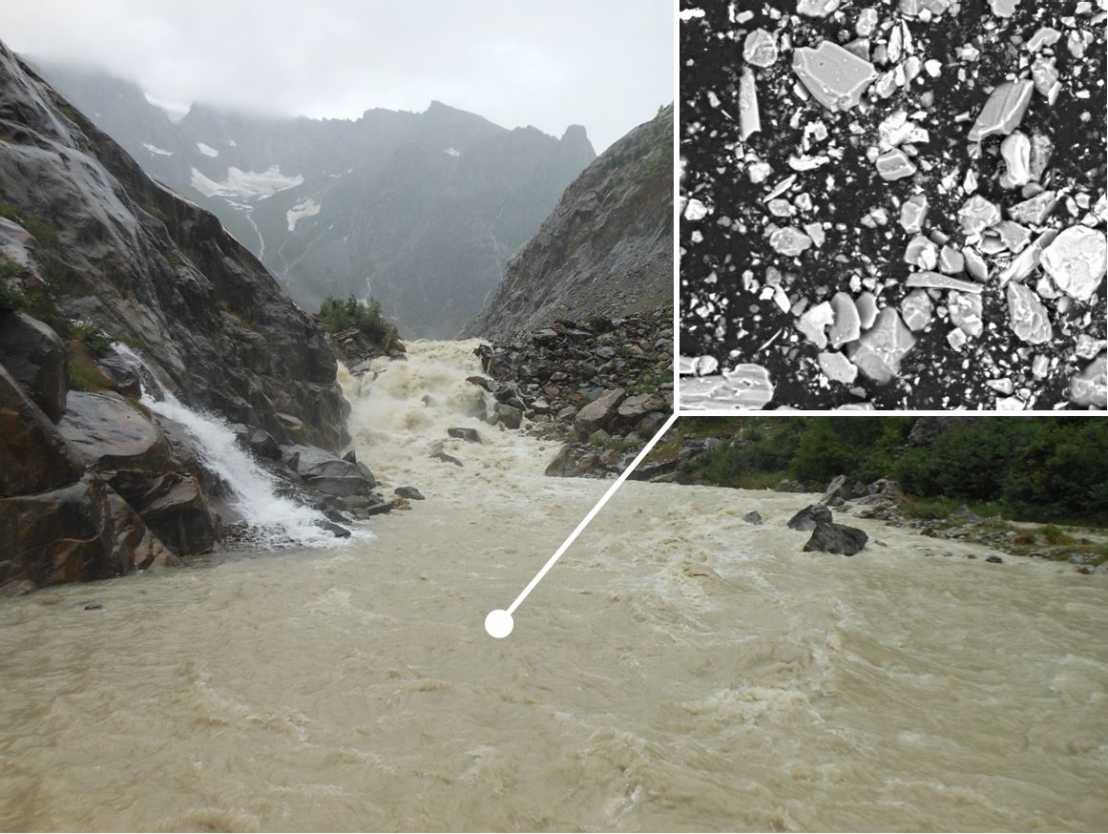
The water driving the turbines in many hydro-electric power plants (HPPs) contains sediment particles. At high flow velocities, hard and in particular angular particles cause hydro-abrasive erosion on turbine parts. This problem is most severe in high-head HPPs equipped with Pelton turbines. Although the application of hard-coatings (tungsten carbide) contributes to increase the times between overhauls, turbine erosion cannot be fully prevented in severe conditions. The consequences are reductions in turbine efficiency and lower electricity generation as well as higher costs for maintenance and spare parts. Hence, the energy efficiency and profitability of HPPs are negatively affected.
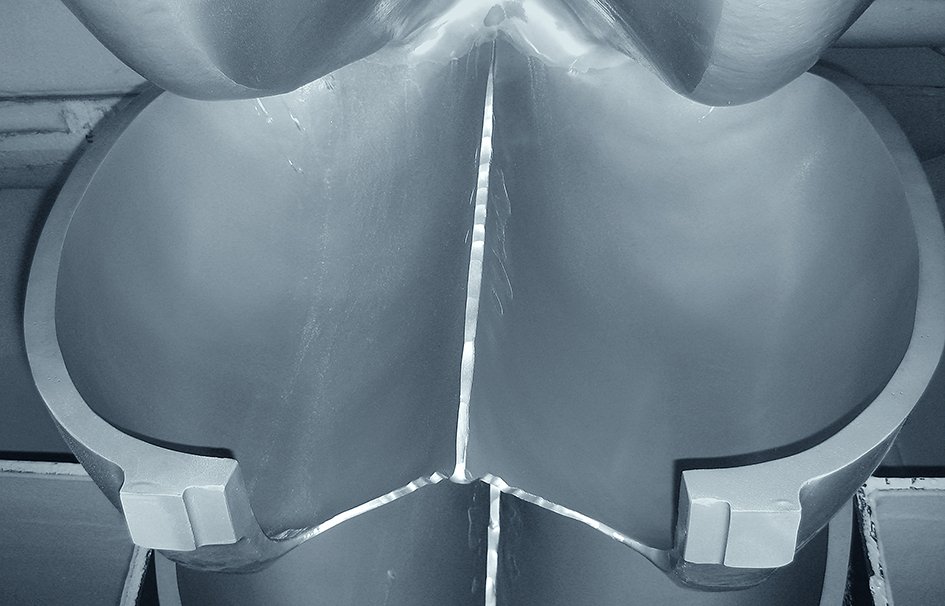
To better understand the relevant processes and to optimize the operation of such HPPs, a pilot project is carried out at the high-head HPP Fieschertal in the Canton of Valais, Switzerland. Quantitative relations between sediment load, turbine erosion and efficiency reductions as well as relevant measuring techniques are investigated until the year 2020. The suspended sediment mass concentration (SSC) and the particle size distributions (PSD) are continuously measured using an innovative combination of five techniques. The erosion on the buckets of the Pelton runners is quantified by optical 3D-surveys and systematic coating thickness measurements before and after each sediment season. The changes in the turbine efficiencies are monitored with periodic sliding-needle measurements as well as evaluation of operation data. The field data are evaluated to (i) further calibrate the erosion prediction model for coated Pelton runners and (ii) optimize the HPP operation, e.g. by temporary turbine shutdowns during floods to avoid excessive turbine erosion.
The project is of key interest for hydropower companies, turbine manufacturers, hydraulic engineers and public authorities. Optimizing the operation of high- and medium HPPs with respect to fine sediment and its consequences improves their cost effectiveness and increases the energetic efficiency. Hence, the pilot project contributes to Switzerland’s ‘Energy Strategy 2050’.
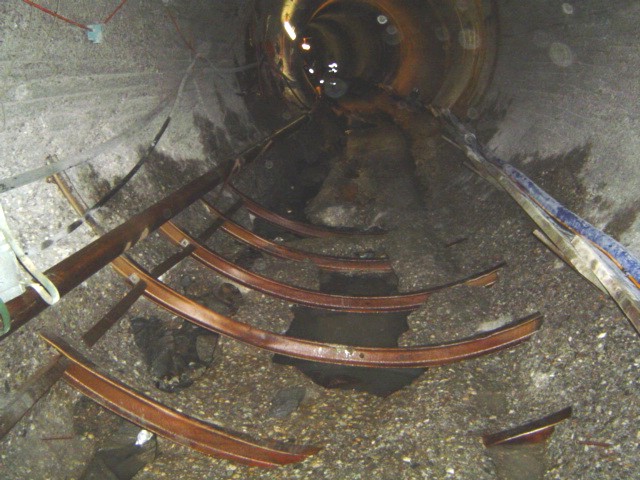
Worldwide thousands of dams impound rivers to create storage volume and thus regulate water for power plants, provide flood retention volume, ensure water supply for human consumption and make watercourse segment navigable. Due to the continuous sedimentation, the storage volumes reduce. Consequently, the versatile functions of the reservoirs and the operating safety or even the dam stability are endangered. Sediment bypass tunnels (SBTs) are an effective measure against reservoir sedimentation by diverting sediment-laden water past the dam and allow for re-establishing the natural sediment continuity. However, high-speed sediment-laden flows in SBTs can cause severe invert abrasion, which may reduce their cost-effectiveness and endanger the sustainable use of water and energy supply.
Abrasion prediction is essential for design life estimations, selection of adequate lining materials and cost-effectiveness analyses. Different mechanistic abrasion prediction models stemming from laboratory experimentation are available, whereas their applicability to field is scarcely investigated. Therefore, this project aims at calibrating and enhancing the abrasion models by quantifying abrasion resistance of various invert materials at three Swiss SBTs. As a prerequisite, sediment transport, hydraulics and hydroabrasion are monitored at Solis, Pfaffensprung and Runcahez SBTs. Furthermore, a Swiss Plate Geophone System for bedload monitoring is calibrated. The findings of the present pilot project will contribute to optimization of design and operation of SBTs as well as other hydraulic structures facing hydroabrasion. Finally, this project aims at contributing to a successful realization of the Swiss ‘Energy Strategy 2050’.
The present project is strongly linked to an on-going BFE project entitled RESEMO - Reservoir sedimentation, management and operation at the case study reservoir Solis.
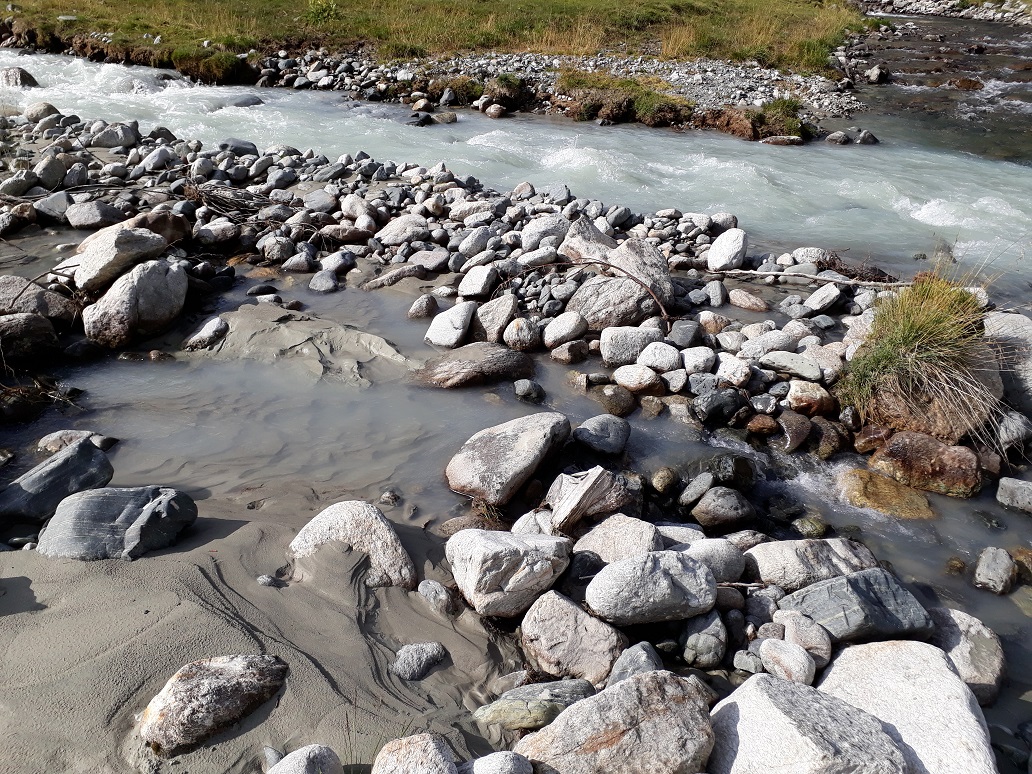
Mountain streams may transport fine and coarse sediment which are a challenge in the planning, operation and maintenance of run-of-river hydropower plants. Coarser sediment particles (stones, gravel and sand) are excluded from the turbine water at the intake and in sand traps. Finer sediment particles (silt and clay), however, remain in the water and cause hydro-abrasive erosion on turbine parts. Small hydropower plants (SHPP) with high heads are particularly affected due to the high velocities inside Pelton turbines. Hydro-abrasive erosion reduces turbine efficiency and hence production. Together with increased costs for repairs and spare parts, the energy efficiency and the cost-effectiveness of SHPPs are negatively affected.
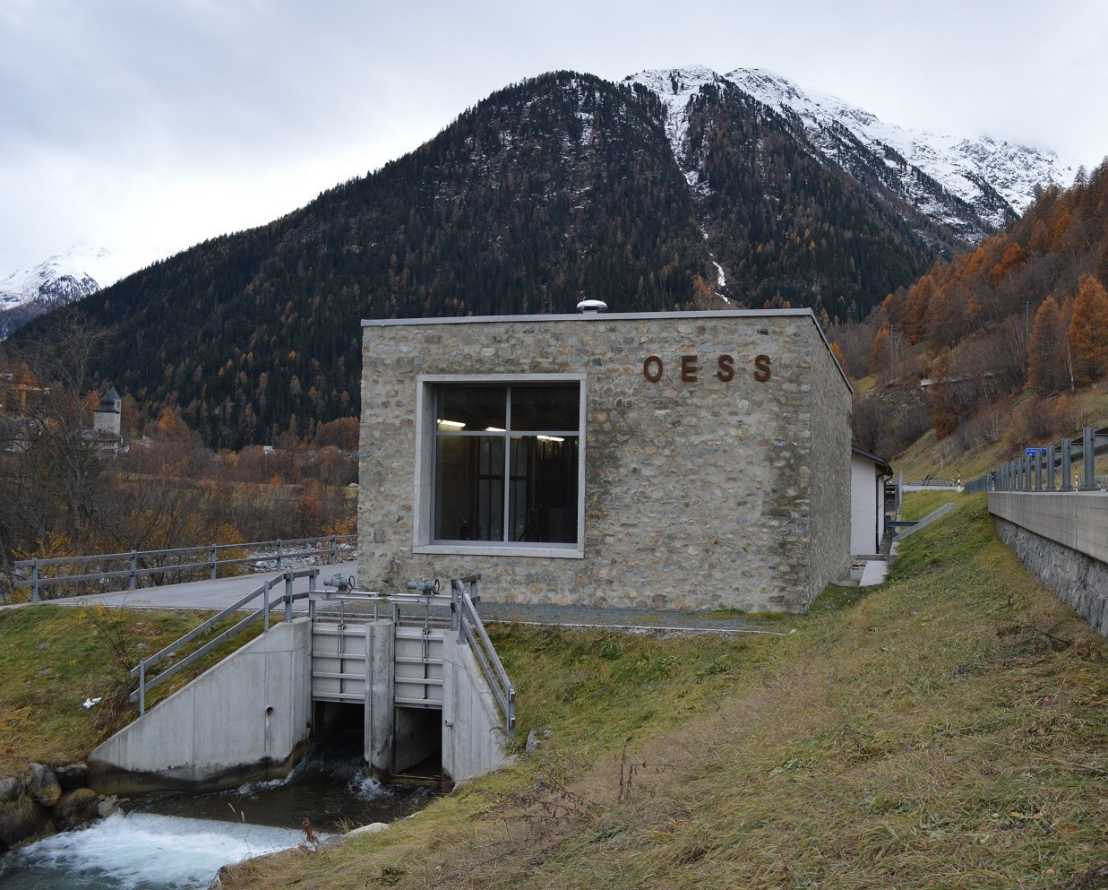
The problem of hydro-abrasion can be mitigated by increasing the resistance of the turbines by hard-coatings and/or by reducing the sediment load in the turbine water. For the latter, a good design and operation of the sand traps as well as a real-time sediment monitoring are important. These topics are addressed in a case study at the SHPP Susasca in the Canton of Grisons, Switzerland.
To quantify the sediment load, the suspended sediment concentration (SSC) in the river and the turbine water are continuously measured using several instruments. With respect to the sand trap, the flow field, the sediment deposition, the flushing procedure and the sediment exclusion efficiency are investigated. In the power house, the erosion on the Pelton runners is quantified periodically. The reductions of turbine efficiency are evaluated from operation data. Based on the collected data set, the relations between sediment load, turbine erosion and efficiency reductions are analyzed to contribute to the development of prediction models. Moreover, measures to improve the sand trap efficiency and the SHPP operation with respect to turbine erosion and its consequences are developed.
In combination with investigations at other HPPs, this project contributes to improve the cost effectiveness and energetic efficiency of high- and medium head HPPs. Therefore, this project contributes to Switzerland’s ‘Energy Strategy 2050’.
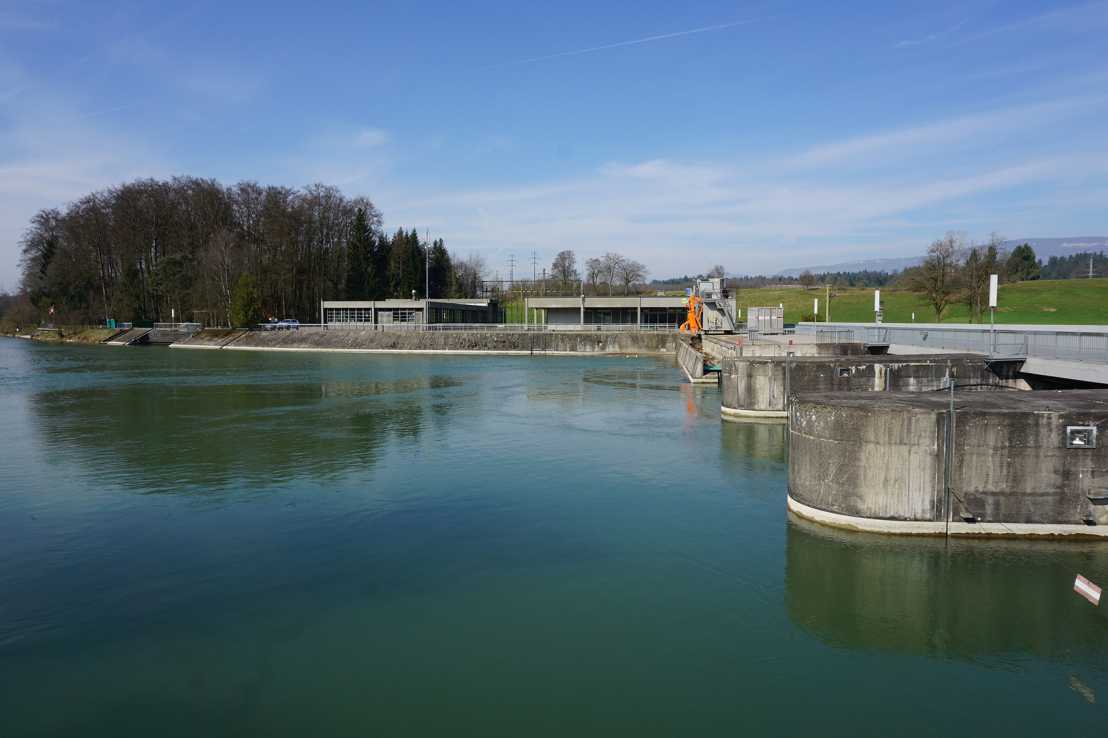
The run-of-river hydropower plant (HPP) Bannwil is a block-unit power plant at the River Aare in Switzerland. The gross head amounts to 5.5 – 8.5 m depending on up- and downstream water level with a design discharge of 450 m3/s. The three bulb turbines have an installed capacity of 28.5 MW and an average annual production of 150 GWh. Downstream of the Bannwil HPP there are nine further power plants and two nuclear power plants with water abstractions for cooling significantly affecting the flow regime.
The target fish species are salmon and barbel. For the upstream migration, a fish pass is installed, which has to be renewed until 2020. Downstream migrating fish are routed through the turbines or over the weir in case of flood events. Protection and bypass systems have to be installed until 2025.
Within the FIThydro project, 250 fish will be equipped with radio-telemetric tags. Their migration routes in the vicinity of the power plant will be observed for 2 years. The fish behaviour will further be observed with sonar camera systems at specific locations (e.g. in front of the intake rack). Operational measures for a safe downstream migration will be considered as well. To this end, VAW is conducting ADCP measurements of the velocity field and will set up a 3D numerical model to compare different alternatives. Furthermore, the installation of a fish guidance structure with vertical bars and an adjacent bypass system will be considered.
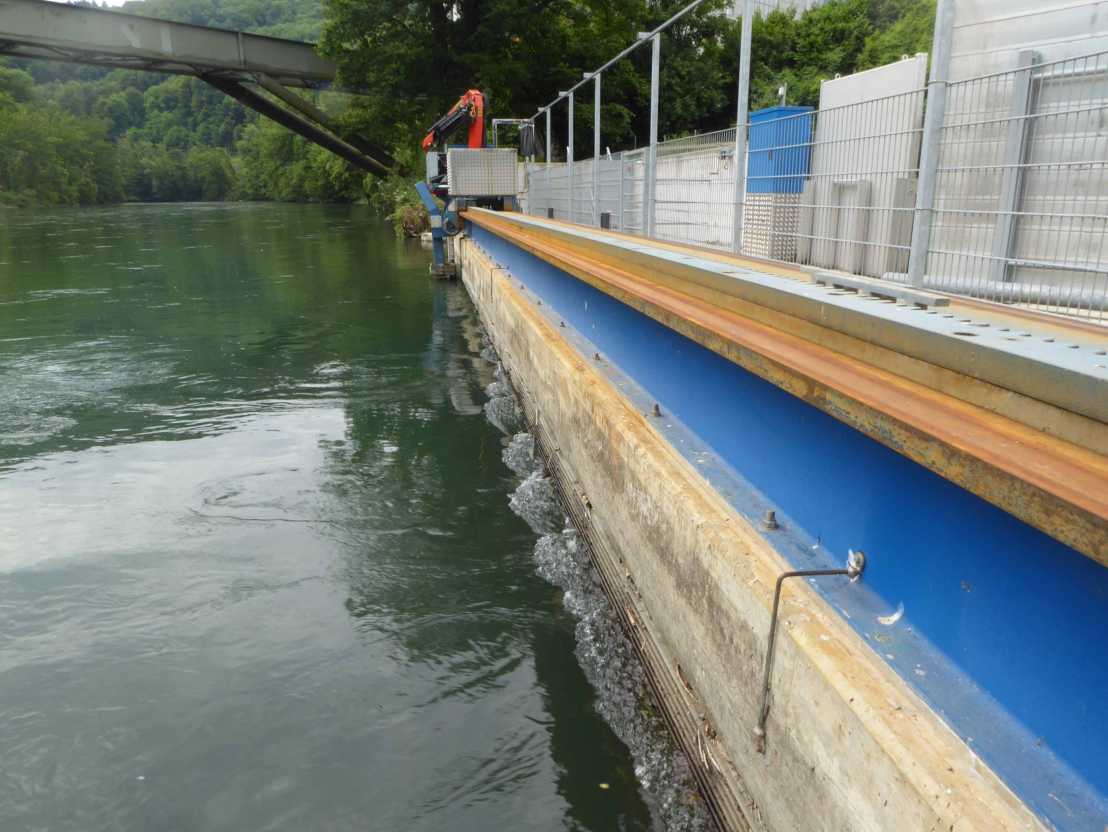
The residual flow hydropower plant (HPP) Schiffmühle is equipped with a bevel gear bulb turbine. It uses a gross head of 2.97 m and a design discharge of 14 m3/s. A capacity of 285 kW is installed, which makes it possible to produce 1.9 GWh a year. Thereby approx. 430 households can be provided with renewable electricity. The 400 m long side weir is positioned parallel to the headrace channel of the main HPP. The HPP Schiffmühle is equipped with a natural fishway and a technical fish ladder (vertical slot) for upstream migration, as well as over a fish downstream migration facility.
The HPP was equipped with a horizontal bar rack bypass system in 2013. The rack is positioned parallel to the main flow to have a lateral intake. The bars have rectangular profiles and a clear spacing of 20 mm. The approach flow velocity at design discharge is 0.5 m/s. At the end of the rack there is a bypass with 3 openings in a vertical chamber in different water depths (close to the bottom, central and close to the surface). Due to the adjacent pipe bypass, the fish have the possibility to migrate downstream to the residual flow reach.
Within the scope of the FIThydro-project the velocity field in front of the HPP Schiffmühle will be investigated with ADCP measurements. To quantify the sediment balance, the bed load transport in the vortex tube connecting the power canal of the main HPP with the residual flow channel will be monitored. In addition, the flow conditions and the aquatic habitats will be modelled numerically. To monitor the success of the fish migration more than a thousand fish will be marked with PIT-tags. To track their swimming paths, all upstream and downstream migration corridors are equipped with RFID antennas.
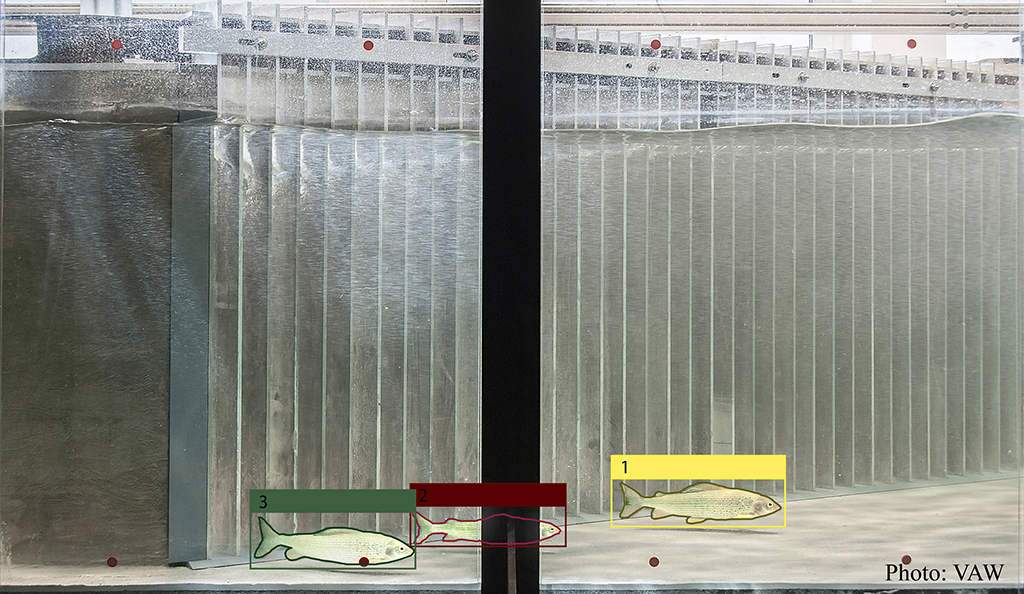
Fishes are in risk of injury during their downstream migration via hydro-power plants. The knowledge on fish behavior in the near field of fish guidance structures is of importance for both the evaluation of their effectiveness and their optimization. However, up to now, fish-observations have been only qualitatively realized. In the first part of this project an automated image-based 2D Fish-Tracking Module had been developed. It is able to detect either the path of a single fish or the midpoint of a fish schooling from video recordings obtained during etho-hydraulic laboratory flume experiments (Fig. 1). The module is mainly based on the Computer Vision System Toolbox™ of the commercial software MATLAB. However, an unambiguous separation of the full path of each fish in a schooling still remains challenging. In the second part of the project, the 2D Fish-Tracking Module has now successfully been automatized, further developed, and applied to a large data set of different fish species (Fig. 1). The newly developed tool is now ready to be applied for analyzing extensive data sets.
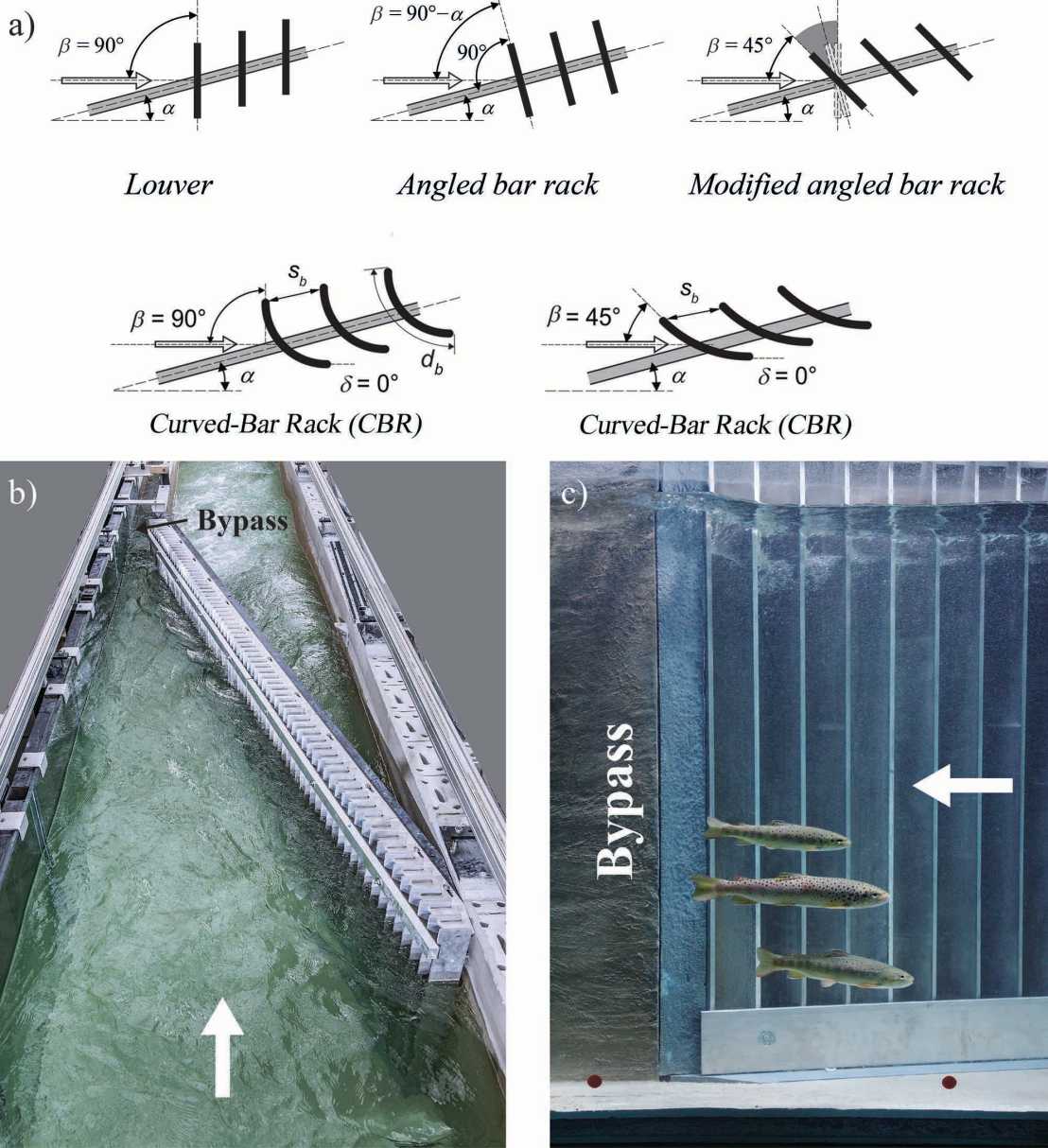
The extensive expansion of hydro-electric power plants all over the world in the past decades has impaired natural river systems and aquatic habitats regarding their flow diversity and the passage of sediments and migrating organisms. Within the EU, the European Water Framework Directive (WFD) enforces a sustainable river restoration. In Switzerland, the strategic plan for the future use of energy includes a gradual nuclear phase-out. In consequence, clean and sustainable hydropower will gain in importance and will likely increase its share of currently 56% in the Swiss energy market. Additionally, the newly revised Swiss Water Protection Act (WPA) demands the revitalization of water bodies compromised by manmade structures by 2030. This includes the restoration of the ecological continuum and thus the unharmed passage of migrating fish and other organisms.
Run-of-river plants pose an obstacle to migrating fish. While various technologies for upstream fish migration have been developed to the state where individual systems can be implemented at existing or new hydropower plants, downstream migration problems have been neglected and have to be addressed now as an urgent matter to stop the decline in the fish population.
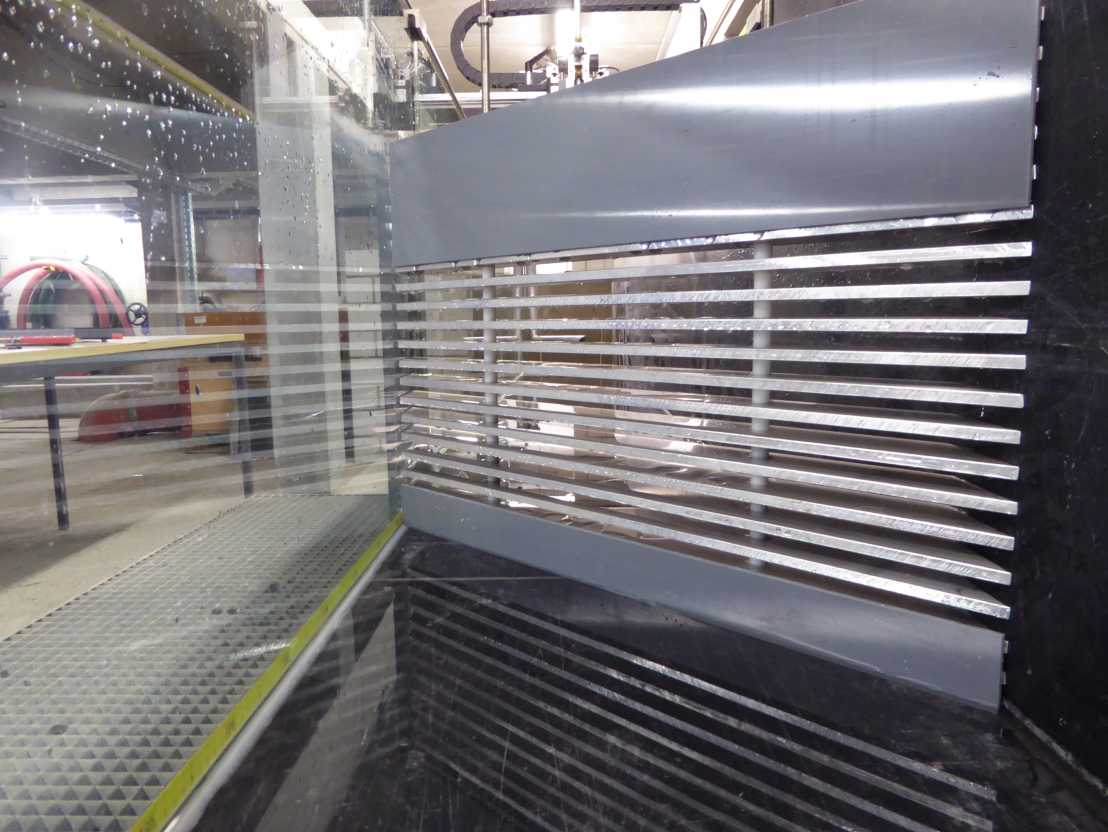
For this reason, the project “Fishfriendly Innovative Technologies for hydropower” (FIThydro) was launched as part of the Horizon 2020 EU Research and Innovation program to promote the development of innovative technologies for sustainable, fish-friendly operation of hydropower plants in Europe. The Laboratory of Hydraulics, Hydrology and Glaciology (VAW) of ETH Zurich is part of the interdisciplinary research team consisting of various European institutes and participants. VAW is involved with two projects developing fish guiding technologies based on physical model investigations. To this aim, two kinds of models are used: small-scale models to investigate the hydraulics of fish guidance structures and a large-scale, so-called ethohydraulic model allowing to study the fish swimming behavior in live fish experiments in the laboratory. While one PhD study focuses on horizontal bar racks currently mainly installed at smaller power plants (QD < 100 m3/s), the second PhD study focuses on vertical bar racks with an optimized bar shaped for the application at medium to large hydro power plants. The objective is to better understand the interaction of fish behavior patterns with different flow conditions created by means of technical measures. Additional field studies in Switzerland and other parts of Europe will be carried out to calibrate the models and validate the performance of existing fish guiding technologies. The research project contributes to the sustainable and efficient usage of water power in Switzerland and Europe.
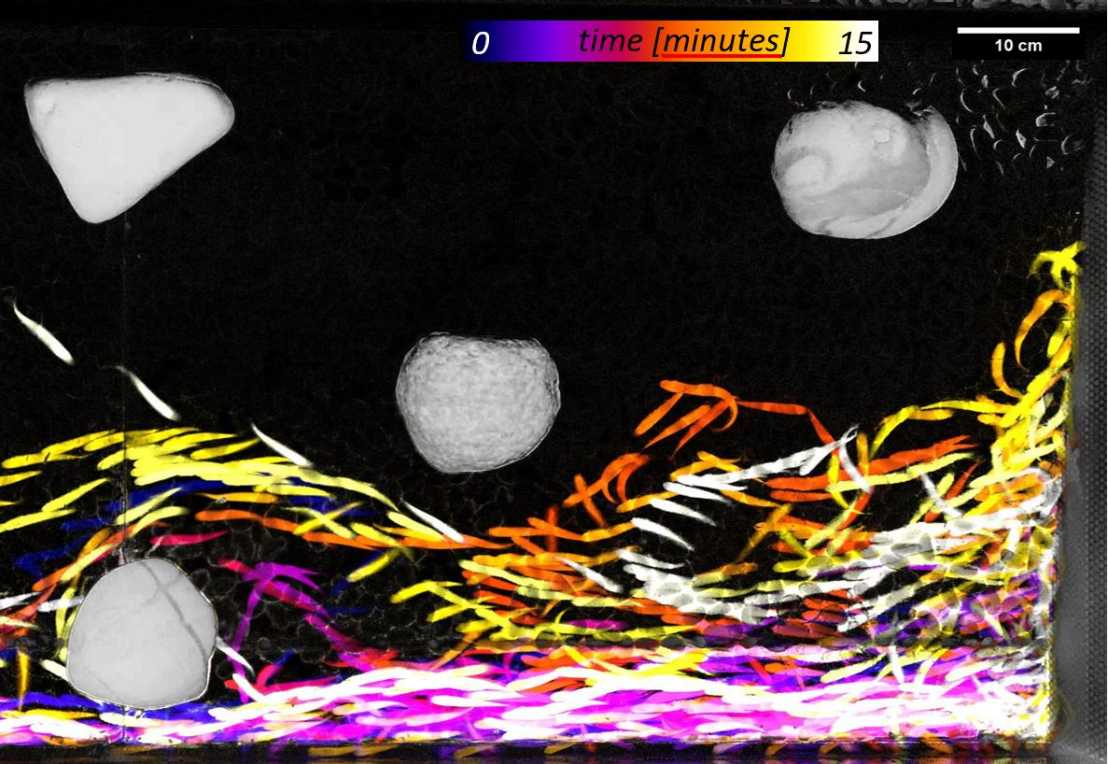
High-head storage hydropower is a crucial energy storage component buffering sub-daily energy demand fluctuations. However, the operation of such facilities imposes severe fluctuations in water discharge and temperature downstream of the dam, known as hydro- and thermopeaking, respectively. These fluctuations threaten riverine fish by disturbing habitats, which may lead to forced drift or stranding, particularly of early life stages.
How do young fish respond to such water discharge and temperature fluctuations? Can they identify signs of flow alteration and react to avoid being stranded or forced downstream with the current?
Using state-of-the-art animal-tracking tools and innovative experimental designs in the flume we aim to determine the behavioural and physiological responses of brown trout parr to these temporal flow and temperature disturbances. This will be achieved by quantifying fine-scale movement behaviour of individual fish in relation to spatio-temporal changes in the flow-field and across distinct thermal gradients. Our findings will be important to provide science-based evidence to discuss hydropeaking mitigation and, therefore, balance societal needs with the preservation of freshwater ecosystems.
This project is a collaboration between the Stocker Lab and VAW.
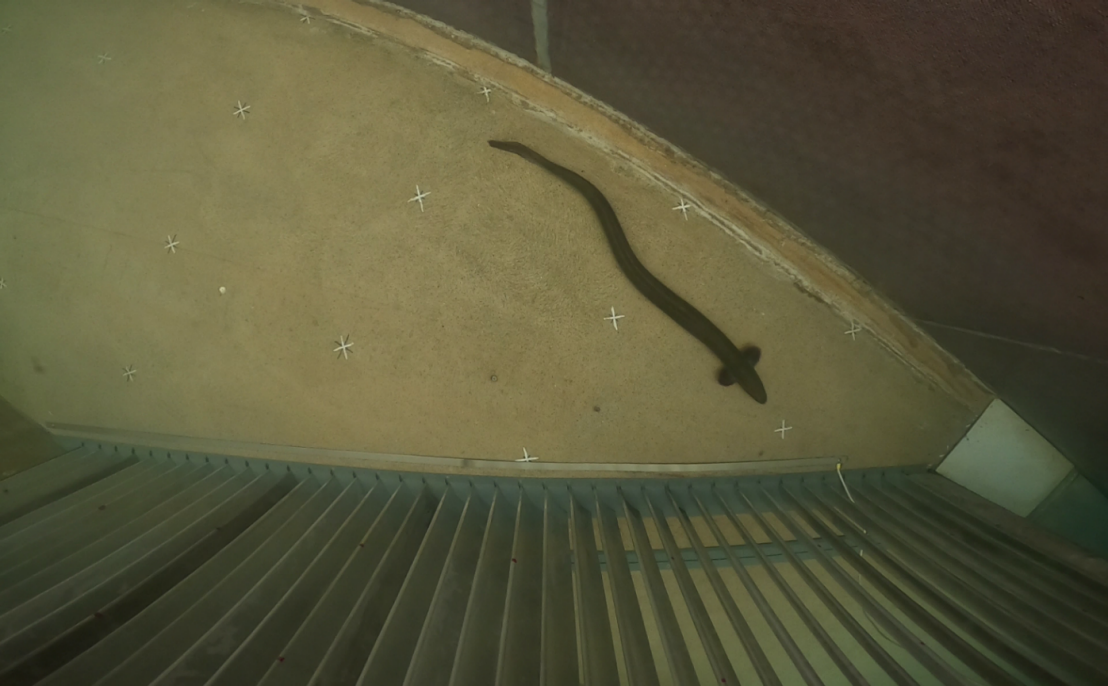
Hydropower plants interrupt the longitudinal connectivity in river networks, which hinders fish movement and puts downstream migrating fish passing through turbines or over weirs at risk to sustain considerable injuries and mortality. Especially the European Eel (Anguilla anguilla) is strongly affected with mortality per plant varying between 25% and 100%. Among other factors, this has led to a decline in fish stocks in recent years. The revised Swiss Waters Protection Act demands the revitalization of water bodies compromised by manmade structures by 2030. This includes the restoration of the ecological continuum and thus the unharmed passage of migrating fish and other organisms. While various technologies for upstream fish migration have been developed to the state where individual systems can be implemented at existing or new hydropower plants, downstream migration problems have been neglected and have to be addressed now as an urgent matter to stop the decline in the fish population.
Two research projects in the scope of the project “Fishfriendly Innovative Technologies for hydropower” (FIThydro), part of the Horizon 2020 EU Research and Innovation program were recently completed at the Laboratory of Hydraulics, Hydrology and Glaciology (VAW) of ETH Zurich. The first PhD focused on horizontal bar rack- bypass systems (HBR-BS) with a narrow bar spacing, which are effective fish protection structures for certain size of fish individuals.
In the second PhD project, a new mechanical behavioral bar rack- bypass system (Curved bar rack - bypass system, CBR-BS) was developed. It is an effective fish guidance structure (FGS) for a large number of species while permitting large bar spacings to reduce head loss and clogging. However, it does not work well for European eels and brown trout (Salmo trutta) as they react poorly to the generated hydrodynamic cues.
For this reason, VAW is developing electrified fish protection racks, with the aim to improve fish protection without compromising guidance at HBR and CBR. To this end, the racks are combined with a low voltage pulsed direct current, to incite an avoidance reaction similar to a pasture fence.
The objective of the experiments carried out at VAW is to improve the understanding of the interaction of fish behavior with different electrical field strength and pulse patterns at various hydraulic conditions.
This research project contributes to the sustainable and efficient usage of water power in Switzerland and Europe.
The present study is a continuation of the BFE project external page EthoMoSt, Etho-hydraulische Modellversuche an elektrifizierten Fischleitrechen.
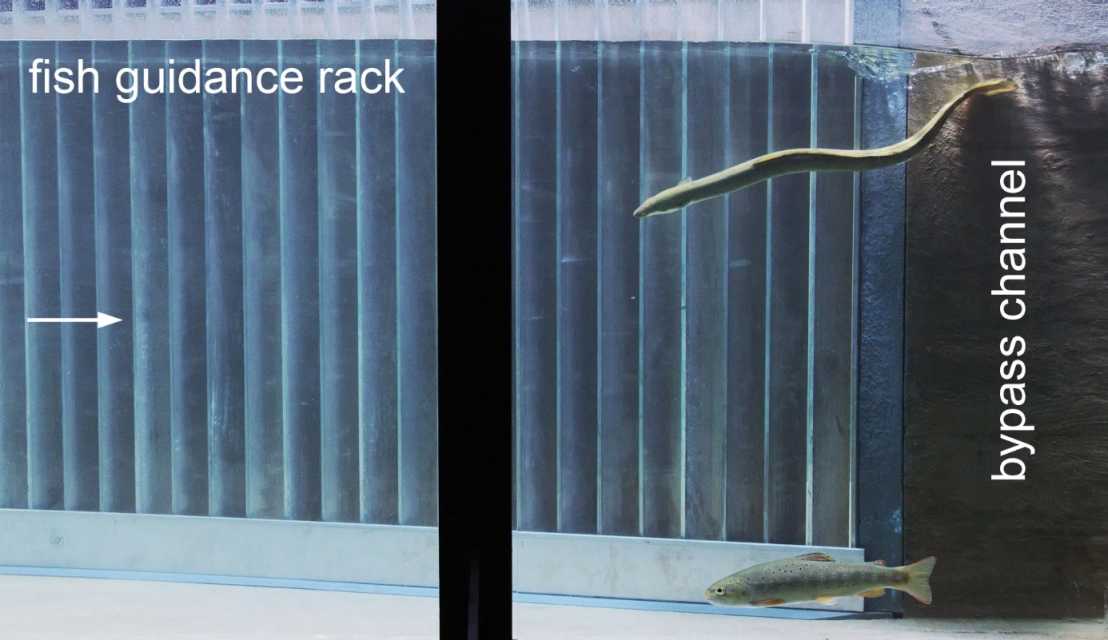
Hydropower plants (HPPs) and other barriers interrupt the longitudinal connectivity in river networks, which among others hinders downstream fish migration and puts fish passing through turbines or across weirs at risk to sustain considerable injuries or even mortality. Among other factors, this has led to a decline in fish stocks. For an efficient restoration of water bodies, several regulations have been introduced including the European Water Framework Directive and the revised Swiss Waters Protection Act and Waters Protection Ordinance. For almost 700 HPPs in Switzerland, downstream fish passage facilities must be upgraded or newly erected until 2030.
For certain fish species, fish guidance racks (FGRs) with adjacent bypass systems (BSs) are effective fish guidance structures for safe downstream fish passage at HPPs and water intakes. However, especially regarding the bypass systems, there are open questions related to its design and operation. This project aims at increasing the fish guidance efficiency of such systems for European key umbrella fish species by systematically investigating current bypass designs and developing an innovative bypass layout. The goal of the latter is to maintain hydropower production by minimizing bypass flow and/or using part of the bypass flow for electricity production. The key fish species are Atlantic salmon, European eel, barbel, European grayling, and brown trout. To reach the project goals, first numerical simulations of various bypass system designs and subsequently ethohydraulic experiments of optimum bypass designs will be conducted at VAW. Furthermore, the effects of sediments and floating debris on the operation of FGR-BSs will be evaluated and technical solutions to counter negative operational effects will be developed.
In parallel to the study of the BSs, hydraulic and biological performance of a Modified angled Bar Rack (MBR) will be evaluated in full-scale conditions at the case study HPP Laudal in Norway for Atlantic salmon by combining detailed fish monitoring with velocity measurements. This will provide crucial information on the behavior of fish under natural conditions when approaching and using guidance structures during their downstream migration.
Overall, this project will contribute to the further development of fish protection and guidance systems. On the one hand, the findings of the study can be used to supplement specific recommendations for the optimal design of fish protection systems and hence contribute to the fulfillment of the requirements set by legal regulations. On the other hand, the fundamental knowledge of swimming skills and behavior of a variety of fish species to hydrodynamics of river flow and FGR-BSs will be extended.
Downstream migrating fish in rivers pass multiple run-of-river hydropower plants (HPPs). Fish tend to follow the main flow and passage through turbine or spillway of the HPPs can result in high injury and mortality rates, which leads to a decline in fish populations. While some alternative fish guiding rack systems have shown promising results, there is a need to develop next generation systems that are cost-effective and easier to operate. In FishPath, we aim to find a completely new way of guiding fish past the water intake of power plants. Via their sensory systems, fish can detect turbulent movements (eddies) in the flow and respond either by avoiding them or by exploiting the eddies for swimming. Fish behavioral response to turbulent eddies will be used to develop guidance systems that create alternative migration pathways for fish around HPPs and other water intakes.
In the multi-disciplinary international FishPath project, led by the Norwegian Institute for Nature Research (NINA), these abilities will be utilized to develop a turbulent eddies based guiding structures for salmon, trout and eel. Therefore, eddies created by different objects (e.g., cylinders, hydrofoils) and how the fish species respond to different types of eddies will be explored. A turbulent Eddies-based behavioral fish Guidance System (EGS) based on a combination of such elements will be designed (Figure). Live-fish tests, and hydraulic measurements of single elements and selected EGS configurations will be conducted in etho-hydraulic flumes at VAW to explore the fish-eddy interaction and the guidance efficiency of turbulent eddies. Furthermore, VAW will contribute to the large-scale and full-scale tests of the EGS at the Laxelerator race-track flume at Vattenfall in Sweden and at River Mandal in Norway, respectively. In parallel, VAW will support the numerical study of single elements and EGSs by SINTEF.
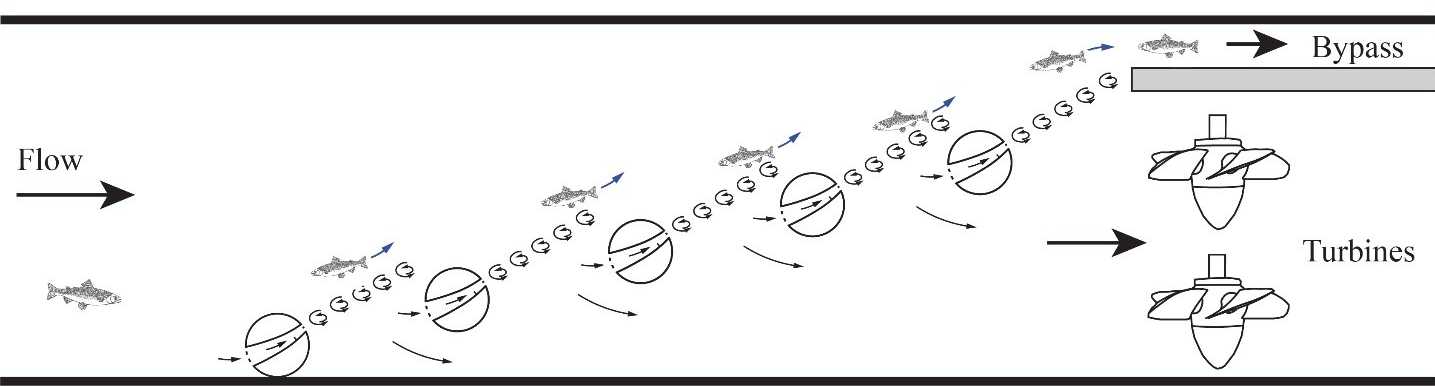
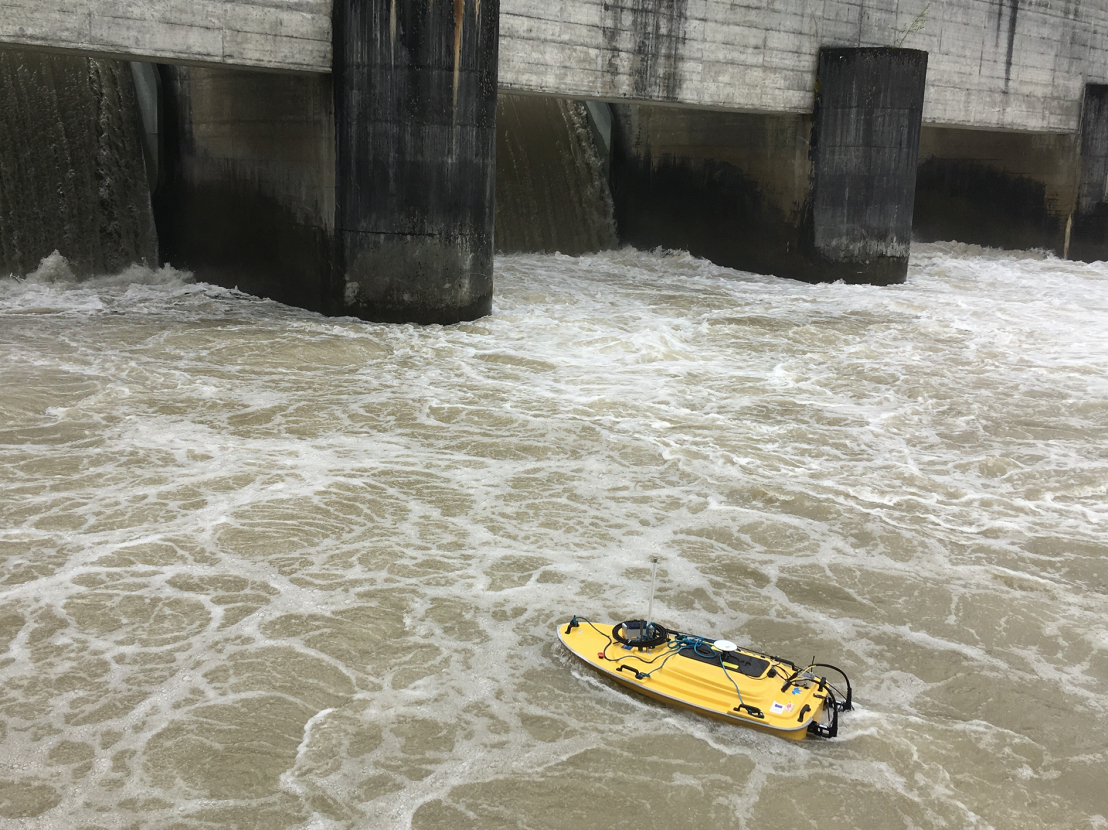
Hydropower plants (HPPs) and other barriers interrupt the longitudinal connectivity in river networks, which among others hinders downstream fish migration and puts fish passing through turbines or across weirs at risk to sustain considerable injuries or even mortality. For an efficient restoration of water bodies, the revised Swiss Waters Protection Act (WPA) and Waters Protection Ordinance (WPO) were introduced in 2000 and 2011, respectively. For almost 700 HPPs in Switzerland, downstream fish passage facilities must be upgraded or newly erected until 2030. One of the promising solutions for downstream fish passage is to use conventional spillways and weirs e.g. in periods of pronounced fish downstream migration. However, the knowledge on fish injury and mortality during weir/spillway passage as well as on the hydraulic conditions in the associated stilling basins is quite limited.
Weir overflow leads to the entrainment of air, which dissolves in water under pressure. Excessive total dissolved gas saturations can lead to gas bubble disease in fish. Such supersaturation and strong pressure fluctuations can potentially cause fish mortality. Therefore, this project aims at investigating hydraulically the safety of fish passage over weirs at the case study low-head HPP Bannwil. To this end, total dissolved gas concentrations, pressure fluctuations and flow velocities are measured and mapped in the stilling basin and downstream reach of the study HPP. This project will underpin the follow-up studies at different HPPs and weirs and may lead to development of new spillway/weir design for multiple fish species.
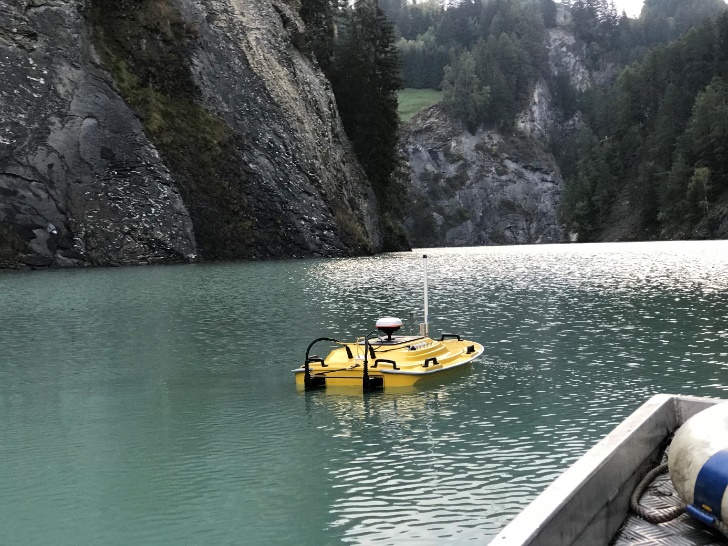
Reservoirs are essential for hydropower production, water supply, flood protection, fishing, navigation and tourism. However, they are subjected to sedimentation on the medium and long term, which affects their functions. Therefore, there is a growing need to mitigate this problem. Sediment Bypass Tunnels (SBTs) are effective measures against reservoir sedimentation by diverting sediment-laden flows past the dam. Their efficiency is expressed by the ratio of bypassed sediment volume to inflow sediment volume. Due to the high cost of construction and maintenance, SBTs are rarely deployed, leading to a lack of experiences, data and knowledge, particularly on the interrelation between reservoir and SBT operations and their impacts on SBT efficiency.
In this project, we aim at a better understanding of the nexus between reservoir sedimentation and management and determining optimal operating conditions. To this end, we conduct field measurements and investigate the hydraulics, sediment transport and deposition processes in the Solis reservoir equipped with a SBT. The bathymetry and high resolution 3D flow velocities in the reservoir are measured using an Acoustic Doppler Current Profiler (ADCP) mounted on a remote control boat with a Real Time Kinematics (RTK)-GPS. The sediment deposition volumes are computed based on annual bathymetry measurements. Bedload transport rates are continuously measured using the novel Swiss plate geophone systems located at the upstream Albula (managed by the WSL) and in the SBT. Based on these data, sediment balances are established and used to analyze the reservoir sedimentation processes. The findings of this study will provide a basis for improving sediment management at reservoirs and contribute to the optimization of reservoir and SBT operations, sustainable use of hydropower as well as the realization of the Swiss ‘Energy Strategy 2050.
The present study is also strongly linked to an on-going BFE project entitled Field monitoring of sediment transport, hydraulics and hydro-abrasion at Swiss Sediment Bypass Tunnels.
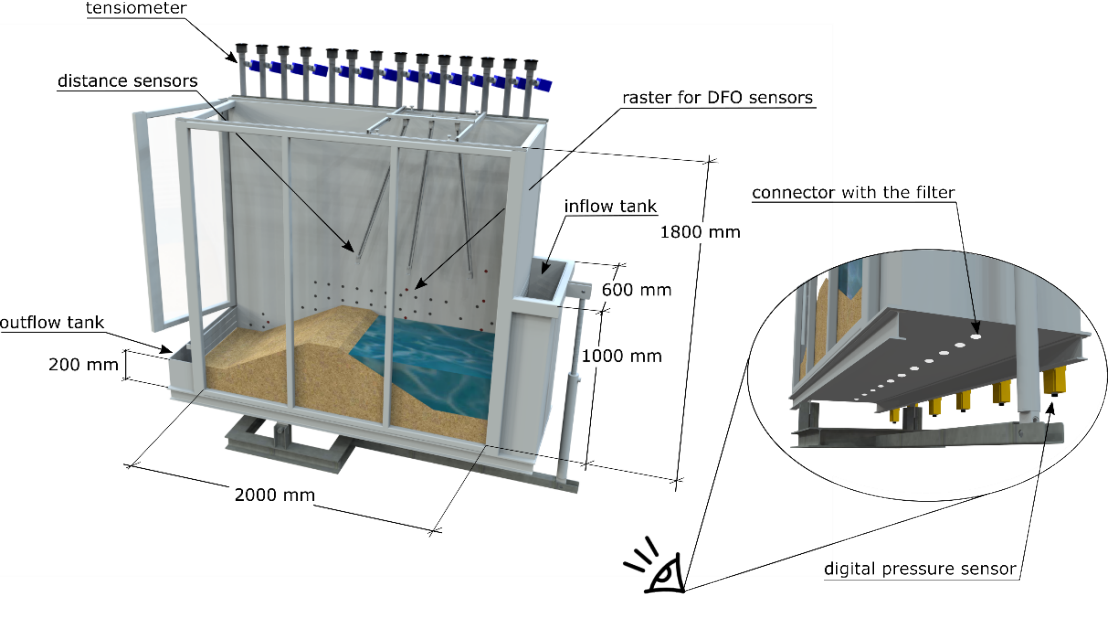
Geohydraulic earthen structures like reservoir dams and flood protection dikes play an important role in water resources and flood risk management. Their structural safety is becoming more crucial due to population growth, increasing urbanization density, and climate change. Depending on the severity of flood events, annual flood damage in Switzerland ranges from several million to billions of Swiss Francs.
One of the most common dam failure causes is internal erosion. The transport of soil particles by seepage flow through or within an earthen embankment forms small channels or “pipes”, which weaken the dam and may lead to failure. The main factor that causes internal erosion is seepage, which can be caused by permeable layers, cracks or fissures, animal burrows, vegetation roots, and human activity (i.e., drilling, vibration).
The most common method to detect internal erosion is by monitoring seepage anomalies through visual surveillance or measurements. Traditional measurement systems are not adequate due to their low spatial and temporal resolution, as geohydraulic structures can extend over hundreds of kilometers, and their failure can occur within a short time (hours) and at a very local scale (meters). Various measurement methods, based on electrical resistivity, self-potential (electrical), or seismicity, have been developed to improve spatial and temporal resolution. However, these methods are not fully automatic and not cost-effective when applied over long distances.
A promising monitoring technology that satisfies spatial and temporal requirements is the distributed fiber optics (DFO) technology. The first application in dams was attempted in Germany, where distributed temperature measured with DFO sensors was used to detect seepage anomalies. Another method involves measuring the distributed pore pressure. Recently, a distributed pore pressure sensor based on DFO technology was developed in Italy. However, this sensor cannot measure effective soil pressure and is not suitable for industrial production, which is essential for field installation.
Following the latest developments, the main objective of this research is to develop a new monitoring system and methodology based on DFO technology to identify, locate, and quantify in real-time seepage anomalies that may lead to internal erosion in dikes and dams. The key aspect of the research is the development of a new DFO soil and porous water pressure sensor that can be produced industrially and is suitable for implementation in real-world projects. The sensor will be calibrated in the laboratory, and later full-scale tested at an outdoor facility in Boretto (Italy) under hydraulic conditions resembling those at prototype structures. The collected data will be interpreted with analytical and numerical models. The developed monitoring system will constitute a valuable tool for risk assessment and provide critical information to river managers for their decisions on flood safety.
Link to the project website: external page https://fibradike.com/

Mass wasting including rockfalls, avalanches or glacier falls can cause impulse waves in reservoirs. Particularly in the case of reservoirs in the periglacial environment, such events are likely to occur more frequently due to changing climatic conditions. For operational matters it is therefore necessary to take the waves caused by smaller, more frequent slides into account for the freeboard dimensioning. The project comprises the investigation of impulse waves in hydraulic model tests and the derivation of suitable design equations.
The project sets the following objectives
- Experimental investigation of the hydraulic processes of the run-up of impulse wave trains on steep slopes in a laboratory channel with a focus on small relative wave heights (Stokes-like waves).
- Quantification of the run-up heights based on the hydraulic characteristics of impulse wave trains.
- Development of an empirical-based calculation approach to reduce planning uncertainties in freeboard design for general reservoir operation.
The main findings include new breaker type prediction criteria for impulsive waves. In addition, a new approach for estimating run-up heights for the first five waves of impulse wave trains was developed. Details on the project and the results can be found in the publication below.

Mass wastings including rockfalls, avalanches or glacier falls can cause impulse waves in reservoirs. For the assessments of the impact of impulse wave events in reservoirs, a manual was developed at VAW. In practice, the lower experimental parameter limitation of the slide impact angle of 30° was identified as too steep in the assessment of individual hazard scenarios. Therefore, this project aims at an extension of the parameter range to shallower slide impact angles starting from 15° for both rockfall- and snow avalanche-like slides. By extending the experimental parameter range, the limitations of the impulse wave manual and thus its applicability for practical purposes will be expanded. The project results contribute to an improved assessment of impulse wave hazards in the context of dam safety.
The project sets the following objectives: (i) experimental investigation of the hydraulic processes of impulse wave generation and propagation at shallow impact angles starting at 15° in a laboratory channel; (ii) quantification of, among others, wave amplitudes based on governing slide characteristics; (iii) comparison with existing computational approaches and, if necessary, development of new approaches.
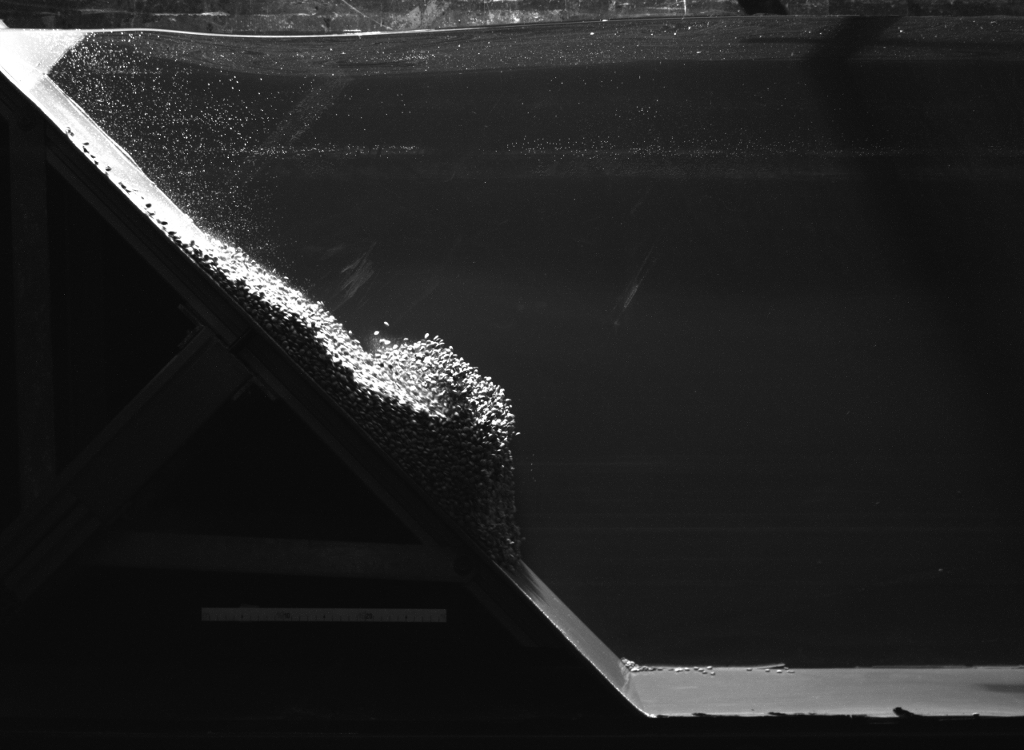
Tsunamis are commonly known for their occurrence in oceans. However, also inland lakes were subject to severe wave inundations in the past. Historic events in Switzerland include Lake Geneva in 563 AD and Lake Lucerne in 1601 AD, when waves with a reported height of several meters impacted the shore and destroyed adjacent settlements. These lake tsunamis may be generated by submarine mass failures, e.g. subaqueous landslides or delta collapses triggered by earthquakes. In the frame of an interdisciplinary SNSF Sinergia research project in collaboration with the external page Institute of Geological Sciences, University of Bern, the Swiss Seismological Service (SED), ETH Zurich, and external page MARUM - Center for Marine Environmental Sciences, University of Bremen, the objectives are to improve the understanding of the underlying triggering mechanisms of lake tsunamis with a focus on lakes in Switzerland as well as the development of a prototype modelling framework for a probabilistic hazard assessment.
The VAW contribution involves modelling the stages of wave generation, propagation, and runup. Laboratory experiments are conducted to gain a better insight into the hydraulics related to wave generation and propagation. These experiments also act as a benchmark for numerical tools. Numerical models are required for simulations at prototype scale and will form an integral part of the probabilistic hazard assessment framework of the overall process chain.